Concrete
Pyroprocessing and Kiln Operation
Published
2 years agoon
By
admin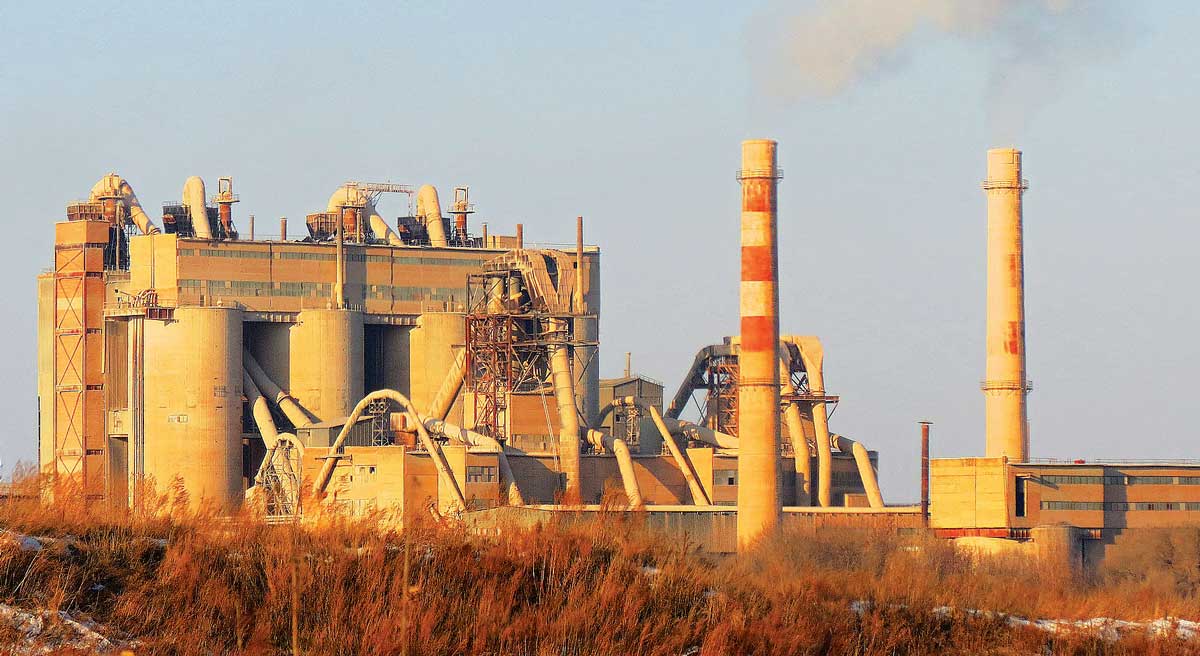
Dr SB Hegde, Visiting Professor, Pennsylvania State University, United States of America, talks about pyroprocessing and the role of preheater, rotary kiln and clinker cooler in the cement manufacturing process. In this two-part series, we will learn all the various factors that aid pyroprocessing.
The composition of Ordinary Portland Cement (OPC) has remained largely the same since the last century and similarly the production process and the chemistry is almost the same. What changed is considerable technological upgradation in the equipment and software for cement manufacture. As a result, more than 12000 tonne per day clinker production from a single kiln has become a reality with latest state of the art fuzzy logic, on line monitoring, advanced operating system with latest data analytics etc. The manipulation and human intervention are relatively reduced, which has resulted in consistent operation, consistent quality parameters both in semi-finished and finished products.
Pyroprocessing in a cement plant comprises a preheater, rotary kiln and clinker cooler. Pyroprocessing section is the heart of a cement plant as actual cement clinker production takes place in kilns. The size of a cement plant is determined based on the pyroprocessing section and the sizes of all other equipment are determined to match pyroprocessing. Cyclones are basic units in a preheater system. Pressure drop and change of temperature of gas across each stage determines the efficiency of cyclones. Introduction of Low Pressure drop (LP) cyclones has brought the pressure drop across each stage to around 50 mm WG from around 150 mm WG in conventional cyclones. This has resulted in more and more plants adopting 5 or 6 stages of preheater. A typical 6 stage preheater with LP cyclones will have a preheater exhaust gas temperature of around 250°C and draught of around 500 mm WG. This in turn led to decrease in preheater fan power consumption.
The reduced temperatures at preheater exhaust contribute to environmental improvement. Cyclone separators are used in preheaters on cement plants to separate the raw material for gases. A very tall preheater means more power is required to operate the plant.
It is always desired for a minimum preheater height to operate the plant economically. Due to the preheater arrangement and layout design cyclones decide the height of the preheater. Pressure drop-in cyclones play an important role in determining the cost of operation of cyclone separators. High pressure drop means more power required to operate the cyclone.
Main KPIs of Clinkerisation
Production rate, ton per dayRun time factor in percentage Specific Heat Consumption in kcal/kg-clinkerSpecific Power Consumption in kwh/ton-clinkerSpecific Cooling Air in kg-air/kg-clinkerSpecific brick Consumption in grams/ton-clinker
The overall process of conversion from raw meal to clinker being endothermic demands a theoretical heat of about 380-420 kcal/kg-clinker. However, the rest of the specific heat consumption as tabulated above constitutes heat losses from preheater exhaust gases, clinker, cooler exhaust gases, preheater dust and radiation losses. Heat loss distribution across different elements can be established through heat balance and process audit of the pyro section. Fuels used commonly to provide heat for the conversion processes are coal, pet coke, and alternate fuels. Alternative fuels like pet rubber tyres, municipal wastes, clinical wastes, greases, rice husks, ground nut husks etc. These materials are being used to become cost effective and to fulfil the condition of Pollution Control Boards while granting the consent to establish and consent to operate the cement plants.
Kiln Operation Guidelines
Based on my experience in cement plant operation, I have jotted down in this paper the important parameters to be maintained for achieving better kiln reliability factor, optimal cost and better quality of clinker production. Cement kiln operation is an ‘art’ once mastered.
Kiln Speed should be such that volumetric loading is within the range 10-15 per cent and heat transfer is maximised. Precalciner kilns generally rotate at 3.5-6 rpm. Under normal conditions, the kiln should be run with as high rpm as possible. Higher kiln rpm improves clinker mineralogy and grindability. Speed control is used to take care of usual kiln disturbances like coating fall down with the other controlling parameters like, fuel rate, preheater fan rpm and kiln feed rate.
Fuel Rate is frequently used as a controlling parameter in kiln operation. Fuel is regulated in the kiln and pre-calciner to maintain required temperature. Oxygen (O2) and carbon monoxide (CO) must be considered first before increasing fuel rate.
Feed Rate is generally maintained in a stable kiln operation. When the control actions, like kiln speed, fuel rate and air control fail or are expected to be insufficient to control kiln disturbance, feed rate is changed as required.
Preheater Fan Speed is varied to fulfil air requirement in kiln system and maintain oxidising conditions in kiln. ID fan speed is not changed frequently in normal kiln operation, unless feed or fuel changed significantly.
Kiln Inlet Analyser gas composition reveals the process (kiln) stability and combustion efficiency. With a good flame in kiln O2 at kiln inlet will be about 1-2 per cent and CO less than 200 ppm, while as it has been observed that an unstable flame may yield in excess of 500 ppm CO with even 3 per cent O2.
Nitrogen oxides (NOx) measurements at kiln inlet gives an early indication of changing burning zone temperatures conditions, before it is reflected in kiln torque trend. It is important to mention that the kiln inlet gas analyser probe position should be inside the kiln to avoid leakage air through the inlet seal to be sucked with sample gas. PC-Gas Analyser is generally installed in the outlet duct from the bottom cyclone to avoid frequent jamming of gas filter due to high dust load in PC outlet duct. Oxygen level should be between 0.5-1.5 at CO less than 100 ppm.
Preheater Outlet Analyser: In preheater down-comer analyser serves both purposes, to measure leakage across the tower and the overall combustion conditions in kiln system. Moreover, it serves as a safety equipment for all critical equipment in upstream gas circuits like ESP, Bag house etc. Oxygen content of 1.5 to 2.5 per cent is considered good at a preheater outlet. Prompt action is recommended if CO increases more than 0.5 per cent.
Lower Cyclone Temperature is considered most important and stable temperature in preheater to control pre-calciner fuel rate. It is generally maintained manually or by PID loop in the range of ±10oC, in the range 850-900oC to ensure calcination between 90 per cent to 95 per cent.
Burning Zone Temperature is monitored by radiation pyrometer. Maintaining constant burning zone temperature means, clinker of constant quality and grindability from a consistent kiln feed. Radiation pyrometer gives a relative value of temperature on the basis of visibility (colour) in the burning zone and can be used as a decisive parameter in stable kiln operation.
Secondary Air Temperature should be as high as possible. It reflects the stability of clinker bed in cooler and the heat recuperation from hot clinker. The higher the best, in the range of 800-1050oC.
Cyclone Cone Drafts: In operation of the kiln, it is a lifeline to monitor all preheater drafts, particularly cyclone cone drafts. Cone drafts in preheater cyclones give an important indication of cyclone jamming along with the other parameters like temperature.
Kiln Back End Temperature indicates the overall stability of kiln operation. It is generally maintained very closely. Variation in kiln back-end temperature indicates either change in burning zone or a change in calciner, hence is of pivotal importance to infer both areas of interest. Back-end temperature is normally maintained at 105oC. Flame shape will determine the flame length and therefore, burning zone length. Flame should be as short as possible, but care should be taken to avoid thermal abuse of refractory due to shorter and one-sided flames.
Kiln Hood Draft should be slightly negative and must be maintained closely between 0 to -2 mm H2O preferably by PID control loop with Cooler vent fan speed. More negative will increase cold air leakages into the kiln through outlet seal and hood, while positive pressures are unsafe.
Cooler Bed Height and Undergrate Pressures: Maintaining constant clinker bed height is a key to stable cooler operation. Undergrate pressure reflects bed resistance and changes with clinker size. To maintain constant Undergrate pressure cooker speed is varied manually or in auto-mode by PID control loop. Constant bed height ensures stable secondary and tertiary air temperatures.
Cooling Air Quantity is maintained to ensure cooling of clinker and heat recuperation from hot clinker from kiln. Specific air usage is generally considered as a key performance indicator of a cooler. New generation coolers can cool the clinker to temperatures as low as 65oC over ambient with a specific air consumption of 1.7 kg/kg-clinker. Generally cooling fans are designed at 2.7 kg-air/kg clinker.
Kiln Torque Load It is a very good indication of the burning zone state, accurate at 70 to 80 per cent of the time. It does not give any major indication for planetary cooler kiln as the load is not always uniform.
Some Important Components of Modern
Kiln System
Main components of kiln system are:
Preheater: Preheaters as name implies serves the purpose of heating raw meal to a temperature where calcination or dissociation of CO2 begins in calciner. Preheater consists of 4-6 low pressure cyclones one over the other. Number of cyclones depends on the natural humidity (moisture) in raw materials, in other words the drying capacity required to dry out raw materials in a raw mill. Five or six stage preheaters are commonly existing in cement plants.
In order to increase heat utilisation in the kiln system, six stage cyclones are as well installed in many cement plants. However, increasing cyclone stages beyond six does not look economic any more, as the quantum of heat saving is not significant to justify it, moreover the increased pressure drop across preheater outbalances the improvements due to additional cyclones.
Preheater Exit Gas Temperature (EGT): Preheater exit gas temperature depending on multiple many factors, however the range for 4, 5 and 6 stage cyclone preheater is tabulated below for reference:
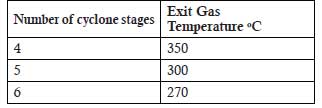
Pressure drops in Preheater ΔP: With the evolution of low-pressure cyclones, it became feasible to go from 4-stage cyclone preheater to 6-stage preheater and harvest more heat in the kiln section. For reference are tabulated the pressure drop values across the 4, 5 and 6-stage preheater.
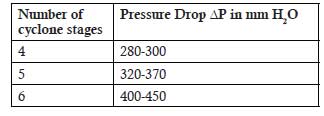
Brick Lining of Cyclones: All preheater components need to be lined from inside with appropriate refractory to save shell/components from heat and to hold heat inside for process use. Refractory castable, bricks and insulation bricks are used in preheaters.
Calciner. Calciner is meant for decomposition of carbonates into reactive oxide calcium oxide. Calcination is an endothermic process and needs heat energy of about 420 kcal. Raw meal is taken in the calciner from the last but one stage of the preheater. Heat for calcination is supplied through secondary firing in the calciner and combustion air is taken from the cooler through tertiary air duct. Various configurations of calciner are existing in modern kiln systems.
Development of calciner have been very significant in multiple in cement industry like:
Burning is more uniform and hence clinker produced is also uniform in quality.Raw meal need not be ground as fine as required for kilns carrying calcination duty.Fuels poorer in quality can be burnt.Raw materials high in alkalies and chlorides could also be used.Throughput capacity of kiln increases 5 times.
Rotary Kilns: Rotary kiln is a rotating cylinder, installed at an inclination of 3.5 to 4 per cent to facilitate material movement. Length and diameter of kiln is decided for the required capacity throughput. Main factors dictating size of kiln are the retention time (25-30 minutes) of material in kiln, degree of filling (10 to 17 per cent) and thermal loading of burning zone (2.8-4.8 x 106 kcal/h/m2).
Precalciner kilns are shortest in length, as 90 to 95 per cent calcination is completed outside the kiln. L/D of three tyre kilns is between 14-17 and for new kilns like ‘Rotax kiln’ it is only 12-13. Kilns are commonly supported on three supporting stations. Each supporting station has 2 rollers and 4 bearings. All rollers are mounted on one fabricated bed plate. Tyre rests on rollers which have an angle of about 30 degrees at the centre of the kiln. Kiln is lined with refractories bricks of 150-250 mm thickness, depending on the diameter of kiln. Basic bricks are preferred in the burning zone; however, 75 per cent alumina bricks are still used for cost consideration. Rest of the kiln is lined with ~ 45 per cent alumina bricks.
Kiln Components
Inlet and outlet seals to prevent cold air ingression in the kiln system.Hydraulic thruster arrangement to prevent slipping of kiln downhill, and to ensure proper floating of kiln.Kiln Drive to rotate the kiln at required speed.Auxiliary Drive for start-stop, maintenance and emergency cooling in case main drive stops/trips.Kiln Burner to insert coal, oil or gas into kiln from the kiln hood. Multichannel burner is used to have a control on flame geometry and use minimum primary air.Nose ring fans to cool kiln outlet tip casting plates nose of kiln.Shell cooling fans are used to cool the shell, to promote coating formation in kiln burning zone areas.
Cooler
Clinker cooler serves two main objectives of cooling clinker from temperature of about 1350oC to the temperature (65-150 0C), where it can be handled by conveyors like pan conveyors, chain, elevators etc. and heat recovery from hot clinker coming out of the kiln. A huge development has happened in clinker coolers designs and types as well. Grate cooler with a take-off for precalciner is generally required for precalciner kilns. Cross bar coolers are used in new plants to achieve cooling efficiencies (>70 per cent) and less maintenance burden. New coolers are designed for the capacity to be handled with the loading of 40-55 tpd of clinker cooled/m2 of grate area. Cooling air requirement is generally designed at 2.2-2.5 nm3/kg-clinker. Either hammer crusher or roller crusher is used to break lumps of clinker before coming out from the cooler. Water spray or air to air heat exchanger is used to cool down cooler vent air before dedusting in ESP or bag filter.
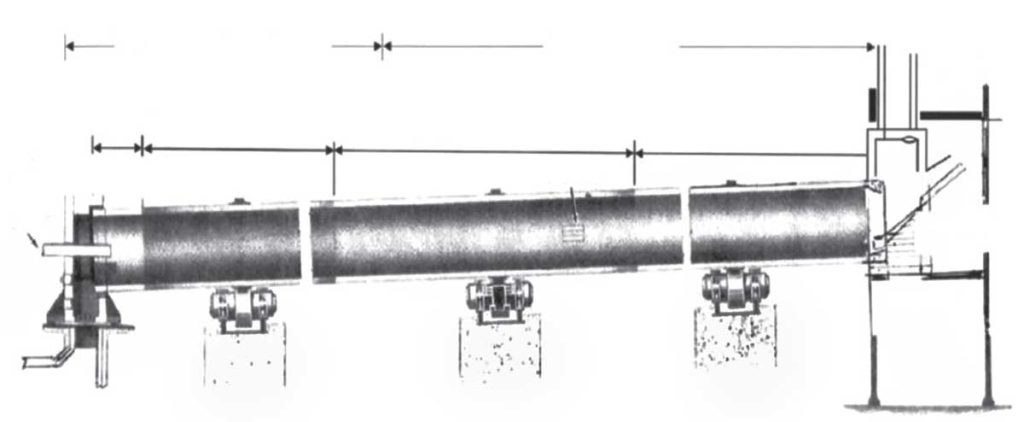
- How to Check or Control Heat Consumption?
Based on my experience the following points should be observed, checked and optimised on day-to-day basis:
Prepare a consistent raw mix: Raw mix characteristics (fineness, quartz content, etc…) and its consistency have an impact on heat consumption. Clinker quality (C3S target) has to be optimised too. - Control oxygen levels in kiln and preheater: While an oxidising atmosphere in the kiln is necessary for a good combustion, the oxygen level has to be minimised. Limiting false air in the kiln line is also beneficial to minimising heat consumption. Reliable gas analysis systems are paramount to master it.
- Optimise fuel combustion: Burners have to be adapted to the type of fuel used by the plant and must be operated within specific parameters (specific impulse and swirl).An oxidising atmosphere is obtained by an adequate draught and a good preparation of the fuel (fineness for solid fuels or atomisation for liquid fuels.
- Master kiln stability: Consistency of kiln feed and fuel dosing is necessary to master combustion. This can be confirmed by clinker free lime results and consistency. A process control system when used appropriately helps minimise heat consumption.
- Optimise cooler efficiency: Optimised airflow distribution, good clinker distribution and size as well as maximised clinker bed depth in the cooler have a beneficial impact on heat consumption; in addition, control loops monitored by a control system optimise operation.
- Master pre-heater and calciner operation: Minimising gas by-pass (flap valves), optimising meal distribution (splash boxes), good material / gas distribution (cyclones efficiency) and managing build-ups are key factors to master pre-heater operation, as well as calciner operation (when there is one).
- Optimise kiln throughput: Operating a kiln at maximum stable throughput minimises heat consumption; identifying current bottlenecks is critical to understand margin for control.
- Maximise kiln reliability factor: Heat consumption is positively impacted by clinker line reliability; reporting all kiln incidents and analysing them during regular team meetings are critical to improve reliability.
- Minimise wasted cement kiln dust or kiln dust loss: Wasted cement kiln dust and kiln dust losses should be monitored and minimised within the clinker quality and kiln process constraints.
- Apply operating procedures with skilled CCR: Operators procedures to start up and shut down the kiln, to manage small kiln stops or to control kiln instabilities have to be written and applied. Kiln operators’ qualification can be improved through skills and knowledge improvement programmes.
Positioning of the Burner
The optimum position depends on many factors. In earlier days, it was a common practice to point the burner a little bit down compared to the kiln axis, in the direction of the charge. This was primarily done to compensate for the tendency of the flame to go upward due to convection and entrainment by the secondary air.
Today, with modern high momentum burners, this is no longer recommended. The jet momentum being stronger, if you point the burner toward the charge, the risk is that the flame will touch the charge. The local reducing conditions would increase Sulphur circulation and increase the risk of coating and blockages in the preheater. Consensus is that high momentum burners should be placed parallel to the kiln axis.
Basic positions would be on the kiln axis, but the burner can also be shifted sideways (still parallel to the kiln axis). Some recommend shifting the burner horizontally away from the charge when using coarse waste fuel to limit the risk that coarse particles would fall into the charge. Similarly, if you use only fine, easy to burn fuel, the burner can be shifted toward the charge to improve heat exchange. As radiation is the primary heat exchange mechanism, the effect is however limited.
Concerning insertion depth, in theory, the further inside is the better. This is to get away from the perturbation of the change of direction of the secondary air and to improve the precooling zone to avoid snowmen in the cooler. There is however a limit due to the length and related weight of the burner and the risk of damage by big pieces of coating falling on the burner. Usual insertion depth would be 50cm-1m inside the kiln. But many kilns operate with the burner just at the limit of the kiln (0cm). Having the burner outside the kiln is generally not recommended.
The burner is usually set at a small angle to the kiln axis and slightly directed on to material. It must be remembered that the material is being cooled by the stream of secondary air flowing over it provided no overheating effects take place in the material; it is thermodynamically desirable to let the flame sweep over it. To prevent the material is getting too hot in to the cooler the final area of the kiln outlet is used as cooling zone, the point of burner is therefore normally pushed forward into the kiln for a distance (0.5 – 1.25 m) in practice it’s found that a very short flame is most suitable for kiln operation in the other hand a very short flame should be used only if a sufficiently thick material crust has been formed.
Significance of Degree of Calcination Percentage (DOC)
The burning of fuel, as well as the residence time of solids depends on the gas flow rate. The calculated gas time varies according to the calculator, from 1.4 to 1.7 seconds, systems with tertiary air flow up to 4 to 5 seconds in total or hybrid flow systems.
Some calciners induce a cyclonic or rotational movement in the gas flow inside the calciner, giving the solids a significantly longer residence time. That is extremely favorable so that a high degree of calcination is obtained, since most of the larger particles will be calcined. If higher calcination rates are reached during the operation, guaranteed kiln production can be exceeded.
The level of calcination will depend mainly:
- temperature inside the calciner
- residence time of the raw meal in the system
- solid gas separationdust circulation effect
- kinetic behavior of raw materials
The calciner system is normally projected for a minimum cancellation rate of 85 per cent being defined by the following equation:
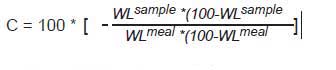
- C = calcination rate percentage
- WLsample = weight loss in fire (calcined meal)
- WLmeal = weight loss in fire (raw meal)
It is extremely important to understand the concepts of the degree of true calcination and the degree of apparent calcination. Degree of True Calcination is obtained when the calcination process was complete, that is, all calcium carbonate (CaCO3) was dissociated into free lime (CaO) and carbon dioxide (CO2).
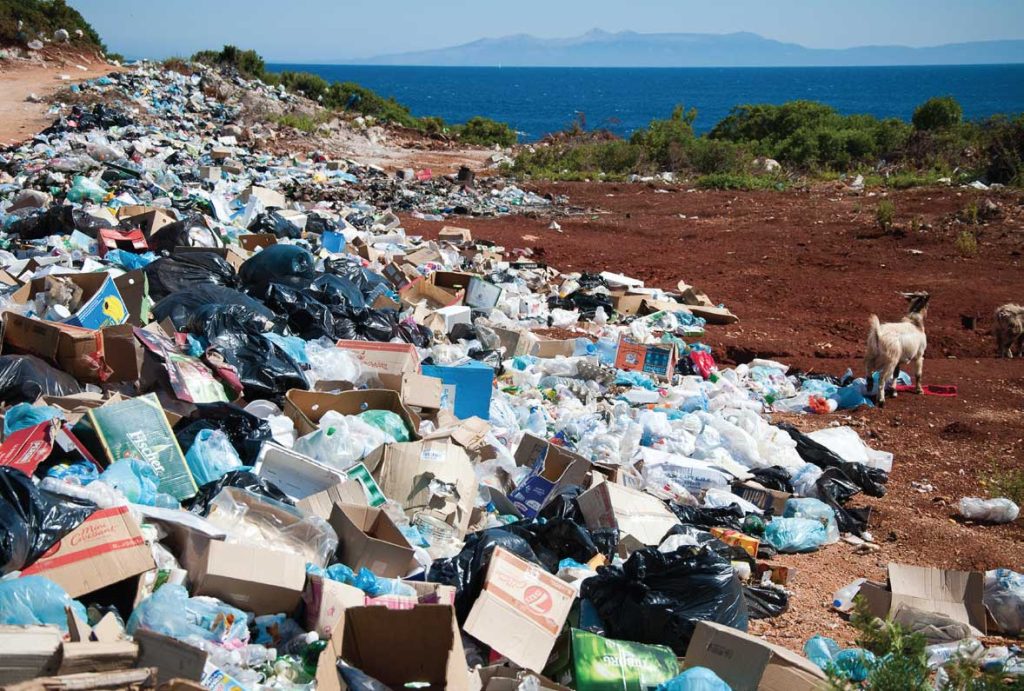
waste, clinical waste, rice husk, ground nut husk, etc.
can make pyroprocessing cost effective and help fulfil
the conditions of the Pollution Control Board.
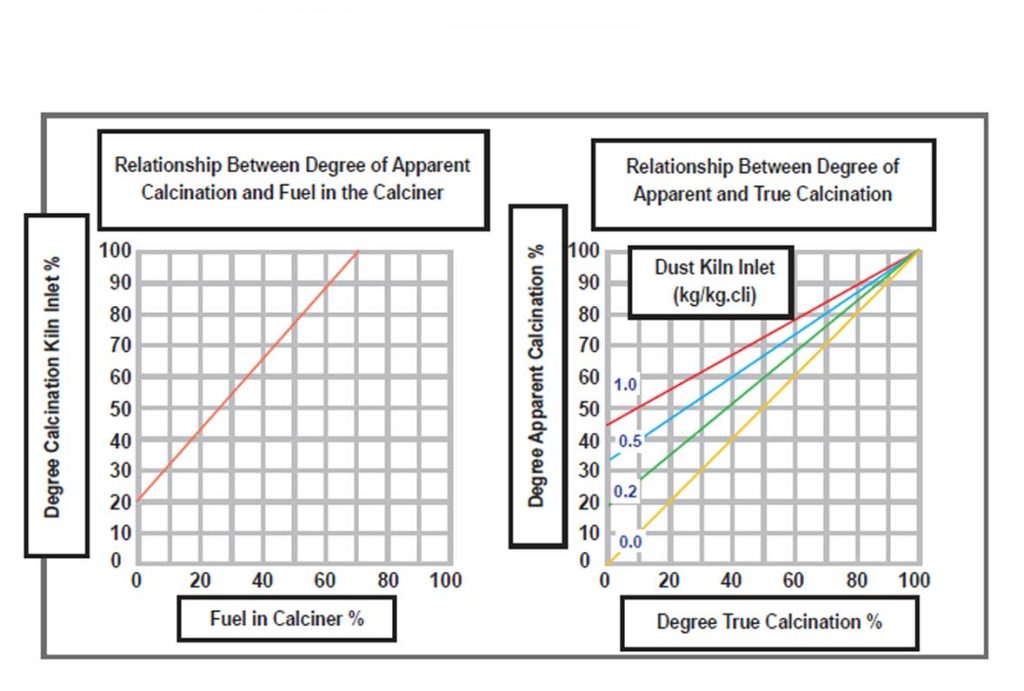
According to this definition, we can consider two extreme cases:
Raw meal – degree of calcination = 0 per cent (weight loss in fire = 35 per cent)
Clinker – degree of calcination = 100 per cent (weight loss in fire = 0 per cent)
In this practice, we never managed to determine the true degree of calcination due to the method of collecting a sample of calcined meal extracted from the cyclone feed chute of the last stage.
As there are dust cycles in the area between the kiln, kiln inlet, gas rise duct and lower cyclone, this sample contains a certain amount of dust that was already present inside the kiln, therefore being ‘contaminated.’ This means that the collected sample contains both powders calcined by the calciner as well as extremely calcined and recycled powder from the kiln. Therefore, the degree of calcination determined with the collected sample was always to have a higher degree of calcination than with the freshly decarbonated hot meal from the calciner.
In conclusion, the degree of calcination determined according to the sample of hot meal collected in the cyclone chute feed the last stage is not a true degree of calcination plus something we call the Degree of Apparent Calcination. This means that the higher the concentration of dust near the kiln inlet area, depending on the number of dust cycles, the greater the degree of apparent calcination.
Whatever the type of calcination used, that is, a separate calciner or a calciner in-line with the kiln, it is mandatory to use a fan to process induction of the system (ID Fan), that is, the combustion gases together with carbon dioxide released in the calcination. On the other hand, in order to obtain an effective control over the secondary and tertiary air flows a control device must be used in at least one of the suction branches, for example, in the tertiary air duct.
For efficient heating of the preheater, a damper installed in the tertiary air duct prevents fresh, cold air from being diverted to the main kiln flame. However, the most important task of this damper is to obtain effective control of the oxygen rate necessary for complete combustion of fuels fed to the calciner Another form of secondary and tertiary air flow control is to install a restriction damper, normally installed in the gas riser duct. This equipment developed by some manufacturers has been used in several factories but costs more than a gate in the tertiary air duct.
ABOUT THE AUTHOR
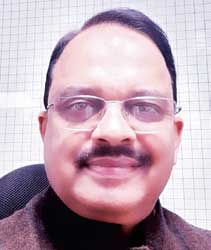
Dr SB Hegde is currently a ‘Visiting Professor’ of Pennsylvania State University, United States of America. He has more than 30 years of experience in cement manufacturing both in India and abroad. He has occupied the ‘Leadership positions’ in reputed major cement companies both in India and overseas. He is also a recipient of ‘Global Visionary Award’ instituted by Gujarat Chambers of Commerce and Industry, Ahmedabad in 2020.
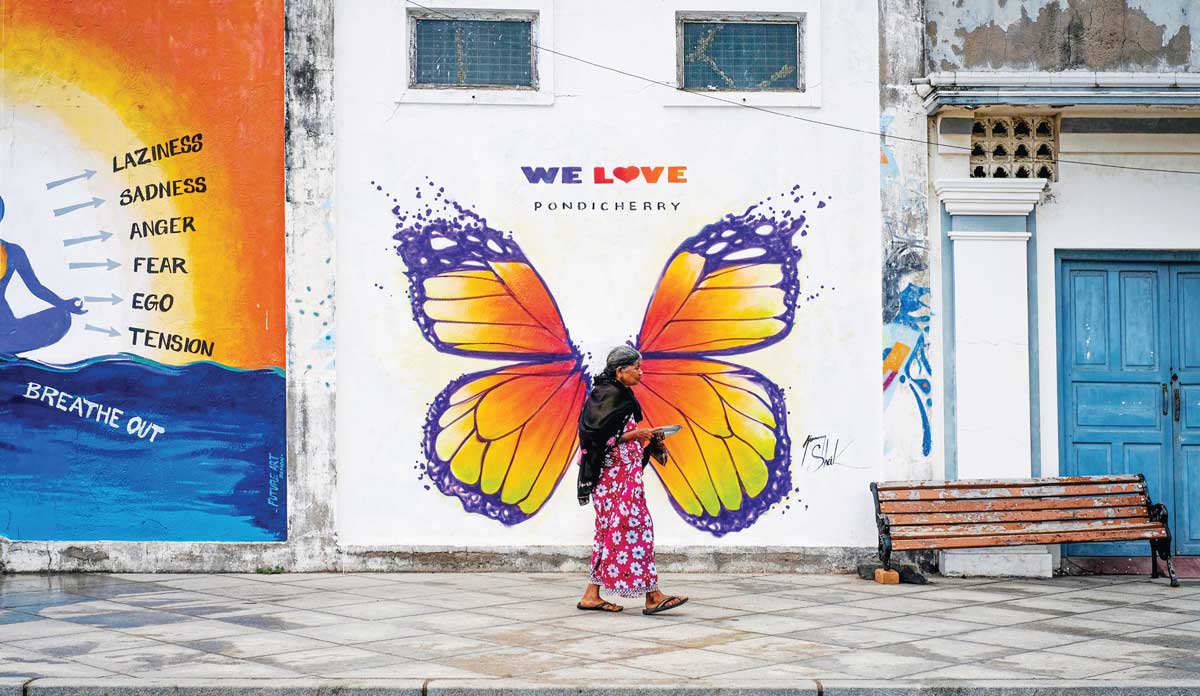
The Concrete in Life Photography Contest 2024-25 by Global Cement and Concrete Association (GCCA) showcased artistic entries, capturing the versatility and beauty of concrete in various settings worldwide.
The annual Concrete in Life Photography Contest 2024/25, organised by the Global Cement and Concrete Association (GCCA), has once again showcased the artistic and functional significance of concrete in daily life. This prestigious global competition attracted over 20,000 entries from professional photographers and amateur smartphone users.
The title of Concrete in Life Photo of the Year 2024-25 was awarded to Henrik Hagerup for his remarkable image taken at Venice Beach Skatepark, Los Angeles, USA. Inspired by the historic 1970s skate culture that repurposed abandoned swimming pools, the image encapsulates the dynamic interplay between concrete and human creativity. As the grand prize winner, Hagerup received a monetary award of $10,000.
Thomas Guillot, Chief Executive, GCCA, who was part of the judging panel, emphasised the competition’s role in highlighting concrete’s essential contributions to infrastructure, architecture and daily life. He noted that this year’s submissions were among the most impressive in the competition’s six-year history, reflecting concrete’s role in shaping urban and rural landscapes. The competition serves as a platform for photographers worldwide to showcase concrete’s aesthetic and functional dimensions.
Hagerup expressed his appreciation for the recognition, stating that his image symbolises how concrete, when integrated with creativity and passion, fosters social connections and opportunities. He emphasised its significance beyond skateparks, extending to communities globally.
The submissions received represented every continent and captured a wide array of concrete applications, from towering skyscrapers and transportation networks to sea defences, bridges and community structures. Many images also depicted intimate human interactions in playgrounds, villages and contemporary architectural designs, illustrating the material’s impact on everyday life.
In addition to the overall winner, the competition recognised four category winners, each awarded $2,500:
- Urban Concrete: Urban Flow by Anvar Sadath TA (Dubai, UAE), featuring the iconic Burj Khalifa.
- Concrete Infrastructure: Structure as Aesthetics by Wentao Guo (Washington, D.C., USA), highlighting a subway station.
- Concrete in Daily Life: Bedok Jetty by Wellington Kuswanto (Singapore), emphasiding the material’s role in everyday settings.
- Beauty and Design: Broken Building by Artemio Layno (Amsterdam, Netherlands), showcasing an artistic perspective on concrete structures.
The People’s Vote Award, determined through a public voting process, was presented to Mohamed Rafi for his image Fluttering Through Life, taken in Pondicherry, India. Rafi received a cash prize of $5,000.
Diane Hoskins, Global Co-Chair of Gensler, a leading design and architecture firm, served as a competition judge and underscored the importance of sustainable concrete practices. She highlighted that while concrete remains a fundamental element in modern infrastructure, ongoing efforts to reduce its carbon footprint are crucial. The competition not only celebrates concrete’s architectural and functional strengths but also encourages industry innovations aimed at sustainability.
PEOPLE’S VOTE – WINNER
Fluttering Through Life by Mohamed Rafi Pondicherry, India
To me, it symbolises resilience, unseen beauty,and how our surroundings shape us in ways we don’t always realise. Street photography is about finding meaning in fleeting moments, and I’m thrilled that this image resonated with so many people.”
URBAN CONCRETE – WINNER
Urban Flow by Anvar Sadath TA Dubai, UAE
“My winning photograph captures the dynamic relationship between concrete structures and the vibrant cityscape, reflecting how concrete shapes our urban experiences. This recognition reinforces my passion for storytelling through photography, and I hope my image inspires others to find beauty in the everyday structures that define our cities.”
OVERAL – WINNER
Venice Beach Skating by Henrik Hagerup Venice Beach, Los Angeles, USA
“My image is a tribute to the way concrete, when embraced by creativity and passion, fosters connection, dreams, and boundless potential not just in skate parks, but in communities across the world.”
CONCRETE INFRASTRUCTURE – WINNER
Structure as Aesthetics by Wentao Guo Washington D.C. USA
“I was captivated by the moment when the transience of transportation runs into the permanence of concrete. Its obscurity becomes its greatest presence: its functionality, its aesthetic and its enduringness. Concrete is such an essential part of our lives and it constructs so many characters of our built environment.”
CONCRETE IN DAILY LIFE – WINNER
Daily Life in Bedok Jetty by Wellington Kuswanto Bedok Jetty, Singapore
“Bedok Jetty holds a special place for me, as I cycle and hang out there every weekend. It inspired me to submit the image of this particular place for the competition, as it showcases a public space made of concrete structure that is actively used by many people engaging in various activities as part of their daily lives.”
CONCRETE BEAUTY AND DESIGN – WINNER
Broken Building by Artemio Layno Amsterdam, Netherlands
“I took the photo at Amsterdam, Netherlands and I was amazed how creative and modern the design of the building is, it looks like it was cut-out and separated in the middle of the main structure, the design is created by a large number of terraces. Concrete is not just used as a necessity in creating structures but it can also be used to showcase artistic works that are amazingly beautiful.”
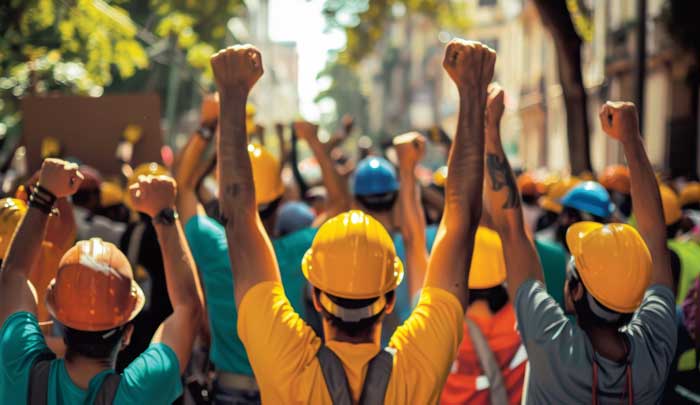
Dr SB Hegde analyses the changing trend in the cement industry as it faces a growing skill gap as ageing workers retire and new talent turns away, threatening efficiency and sustainability.
Cement is the backbone of roads, buildings and cities worldwide. In 2023, the world produced 4.1 billion tonnes of cement, and that number could grow to 4.7 billion tonnes by 2030 (US Geological Survey, 2024). However, there’s a big problem — the cement workforce is ageing and not enough young workers have the skills to operate modern machines or meet new environmental standards. This skill gap — the difference between what the industry needs and what workers can do — is growing quickly. It’s a hidden threat that could slow down construction projects and
hurt economies.
Cement drives development worldwide, but countries like India are feeling the pressure the most. In 2022, India produced 410 million tonnes of cement, making it the second-largest producer after China (Statista, 2024a). With major infrastructure plans for roads and railways, India needs skilled workers more than ever. However, many cement plants are struggling to find workers who understand modern tools or environmentally friendly methods.
This article explores why this skill gap exists, how it impacts the industry, and what can be done to fix it—both globally and in India. With updated statistics and real-world examples, it sends a clear message: cement companies must take action before the problem gets worse.
The hidden crisis
The skill gap in the cement industry is a growing problem that’s not always easy to see. Across the world, experienced workers who have spent decades mastering cement production are retiring. A 2023 report shows that 30 per cent of them are over 50, and new workers aren’t stepping in fast enough, especially in Europe and the US (World Cement Association, 2024).
At the same time, cement plants are becoming more advanced, using technology like smart sensors, automated systems and robots. These systems require workers with technical and computer skills—not just physical strength and basic knowledge.
India is also feeling the heat. With the capacity to produce 690 million tonnes of cement yearly, the industry employs over 1 million people (IBEF, 2024). But according to a 2024 study, 65 per cent of plant managers report not having enough skilled workers to handle modern machinery (CII, 2024). This problem is even worse in southern India, where too many plants compete for limited talent, and low wages push skilled workers to better-paying city jobs.
This skill shortage affects the industry in several ways:
- Higher maintenance costs: Without skilled workers, machines break down more often.
- Reduced cement quality: Poorly operated machinery affects the consistency and quality of cement.
- Increased production costs: Inefficiencies lead to higher operational costs.
The crisis is reshaping how cement is made and forcing companies to rethink their strategies. If the industry doesn’t tackle this problem soon, it could face severe setbacks in productivity, quality and profitability.
From skill to crisis
Cement production used to rely heavily on hands-on skills. Workers learned through experience—breaking rocks, firing kilns and mixing materials. But today, machines handle most of that work. Around 80 per cent of the world’s cement now comes from dry processes, which require less labour but more technical expertise (Rhodium Group, 2024).
The older generation of workers, who mastered traditional methods, often struggle to adapt to modern systems. Meanwhile, younger workers are not being trained quickly enough to fill the gap. This shift is making the industry less efficient and more vulnerable.
India is a prime example of this transformation. Small cement plants once thrived on local skills and traditional methods. But now, large companies like UltraTech, which produces 79 million tonnes of cement annually, are using advanced technologies like waste heat recovery systems (WHRS) to save energy and reduce emissions (IBEF, 2024).
However, a 2023 survey by the National Skill Development Corporation (NSDC) revealed that only 20 per cent of cement workers in India are capable of handling these modern systems (NSDC, 2024). This lack of expertise leads to several problems:
- Inefficiencies in production: Poorly operated technology slows down manufacturing.
- Reduced sustainability efforts: Without skilled workers, eco-friendly systems like WHRS are not fully effective.
- Higher operating costs: More downtime and maintenance result from improper handling of equipment.
The old craftsmanship is fading, leaving the industry at a crossroads. Without immediate action, this skill shortage could severely impact cement production’s ability to meet growing demands and adopt sustainable practices.
Technology’s double-edged sword
Technology is both a solution and a challenge for the cement industry. Around the world, new tech is making cement production faster, cleaner and more efficient. For example, Cemex tested solar-powered cement in 2023, aiming for eco-friendly production, while Heidelberg invested €450 million in carbon capture technology in Belgium (World Cement Association, 2024). These innovations are part of why the global cement market could reach $686 billion by 2032 (Fortune Business Insights, 2025).
However, the catch is that these high-tech solutions require skilled workers who understand how to operate and maintain advanced systems—not just traditional manual labour.
India is also riding the wave of automation. Cement plants are now getting 20–25 per cent of their power from WHRS, which save energy and reduce costs (World Cement, 2025). When Adani acquired Sanghi Industries for $606.5 million in 2023, it added more advanced technology to its operations. But the problem remains: local workers often lack the skills needed to handle this new machinery effectively.
While technological advancements have helped India produce 375 million tonnes of cement in 2023, they are also creating a divide within the workforce. The industry is splitting into two groups:
- Tech-savvy workers: A small group trained to handle automation, digital systems and advanced equipment.
- Traditional workers: A much larger group lacking the skills needed to work with modern technology.
This divide leads to several challenges:
- Underutilised technology: Many high-tech systems are not fully used due to a lack of trained operators.
- Increased operational costs: Companies spend more on training and maintenance when technology is poorly managed.
- Job insecurity: As automation grows, workers with outdated skills face the risk of being left behind.
The cement industry must find a balance between adopting new technologies and ensuring workers have the skills to operate them effectively. Without doing so, the push for efficiency and sustainability could leave a large part of the workforce struggling to keep up.
Why is the young talent avoiding the cement industry?
Young people are not interested in working in the cement industry. Globally, only about 5 per cent of engineering graduates from the US and Europe consider manufacturing jobs, according to a 2023 report (McKinsey, 2023). They see cement jobs as dirty, boring and outdated compared to high-paying tech jobs with modern offices and exciting projects.
India faces the same problem. Although the cement industry contributes around 6 per cent to the country’s economy, it struggles to attract fresh talent. In 2023, the IT sector hired 1.5 million graduates, while the cement industry managed to recruit only about 50,000 (NSDC, 2024). Cities like Bengaluru, known for their booming tech hubs, lure young engineers who prefer coding jobs over working in hot, dusty cement plants in places like Rajasthan.
The perception problem is real. A 2024 survey revealed that 70 per cent of Indian engineering students described cement work as ‘dull’ and ‘unappealing,’ even though starting salaries can be quite competitive, ranging from `8 to Rs.12 lakh per year (CII, 2024). They don’t see cement as a modern, innovative field with growth opportunities.
Beyond the factory floor
The skill shortage in the cement industry isn’t just a factory problem—it’s a major issue for big construction projects worldwide. When cement plants struggle to find skilled workers, everything slows down, causing costly delays.
In the US, the $1.2 trillion infrastructure plan aimed at upgrading roads, bridges and railways is already feeling the pressure. Cement shortages caused by untrained workers are holding back progress (Fortune Business Insights, 2025).
Africa faces a similar problem. Cement demand on the continent is expected to grow by 77 per cent by 2030. But without skilled workers, meeting that demand will be nearly impossible, stalling economic growth and infrastructure development (World Cement Association, 2024).
India is feeling the pinch more than most. The country’s $14.59 billion infrastructure plan, which includes building highways, smart cities and bullet train projects, heavily relies on cement (IBEF, 2024). One of the most ambitious projects, the Mumbai-Ahmedabad Bullet Train, requires a staggering 20,000 cubic metres of cement daily. But skill shortages are disrupting supply, leading to delays.
The problem isn’t just limited to mega-projects. For example, Chennai’s metro expansion faced major delays in 2023 because local cement plants couldn’t meet demand on time. The skill gap is directly impacting the speed and quality of these projects.
What’s at risk?
- Job creation: India’s construction boom is expected to create around 1 million jobs, but if the cement industry can’t keep up, those opportunities will be lost.
- Economic growth: Infrastructure development contributes significantly to GDP. Delays in cement supply can slow down the entire economy.
- Global competitiveness: If India and other countries can’t resolve this skill gap, their ability to compete on a global scale will be compromised.
Bridging the gap
The skill gap in the cement industry isn’t impossible to fix—new training models worldwide are making a difference. Countries and companies are trying out fresh ideas to build a skilled workforce.
In Germany, a dual education system that combines classroom learning with hands-on factory training is producing 60,000 skilled workers every year (IEA, 2023). It’s a practical approach where students gain real-world experience while studying, making them job-ready from day one.
Companies are also stepping up. Holcim introduced virtual reality (VR) training in 2024, allowing workers to practice operating complex cement plants in a simulated environment. This approach saves time, money, and reduces accidents during training.
India is making efforts too. UltraTech’s skill centres trained 10,000 workers in 2023, focusing on areas like automation, machine handling, and safety (IBEF, 2024). The company is trying to bridge the skill gap by equipping workers with modern technical skills.
Collaborations are also happening. Shree Cement has partnered with IIT Kharagpur, producing 500 skilled engineers annually since 2022. This initiative aims to bring fresh talent into the industry, particularly in high-tech areas.
The National Skill Development Corporation (NSDC) and Ambuja Cement have even bigger plans. They aim to train 50,000 young people by 2029, with a special focus on green technologies and sustainable manufacturing.
Why this matters:
- Reducing the skill gap: If these training programmes expand successfully, India’s 65 per cent skill shortage could be significantly reduced by 2030.
- Improving productivity: Better-trained workers mean fewer machine breakdowns, improved cement quality and reduced costs.
- Boosting economic growth: Skilled workers are essential for completing big infrastructure projects on time, directly supporting economic development.
Sustainability at risk
Achieving sustainability in cement production isn’t just about technology—it’s about having skilled workers who can operate and maintain green systems. The cement industry is responsible for 6–8 per cent of the world’s CO2 emissions, pushing companies to find eco-friendly solutions (IEA, 2023).
In 2024, a plant in the UK managed to cut its emissions by 85 per cent by adopting advanced technologies. But here’s the catch: running these systems needs highly trained workers who understand how to use and maintain them.
India is also striving for greener cement production. Major companies like JK Cement have set ambitious targets, aiming to use 50 per cent biomass fuel by 2030. However, only 15 per cent of the workforce currently has the necessary skills to handle this transition effectively (CII, 2024).
Ambuja Cement’s Concrete Futures Lab is one initiative trying to close the skill gap by training 2,000 workers each year in eco-friendly cement production. But compared to the scale of the industry, that’s not nearly enough.
Why this matters:
- Carbon-neutral goals at risk: India’s target of achieving carbon-neutral cement production by 2050 is in danger if the workforce isn’t adequately skilled.
- Need for better training programmes: Without widespread and advanced training programmes, green technologies will remain underutilised, slowing down the progress toward sustainability.
- Global impact: What’s true for India is true for the world—if we don’t bridge the skill gap, the dream of reducing cement’s carbon footprint may stay out of reach.
- The solution is clear: We need more training centres, better courses, and partnerships between companies, educational institutions, and the government to make green cement production a reality.
The global skill gap
The skill gap in cement manufacturing is a worldwide problem, but it looks different depending on where you are.
In the US, which produced 91 million tonnes of cement in 2023, the main issue is a shortage of workers with digital skills to operate advanced systems (World Cement, 2025).
In Africa, the problem is more basic. The industry lacks trained workers altogether, which could severely impact its cement demand expected to rise by 77 per cent by 2030 (World Cement Association, 2024).
India faces a mixed challenge. Northern cement plants struggle to find workers with the technical expertise to handle modern, automated systems. In contrast, southern plants have a surplus of workers, but their skills are outdated or irrelevant. NSDC trains around 50,000 workers annually, but that’s far too little compared to the rapidly growing demand (World Cement, 2025).
In China, the government takes a proactive approach, training 200,000 workers each year through organised programmes to support its massive production of 2.1 billion tonnes in 2022 (Statista, 2024b).
Why this matters:
- India’s demand boom: Cement demand in India is projected to increase by 42 per cent by 2030, but without the right skills, meeting that demand will be difficult.
- Learning from others: Countries need to collaborate, sharing training models and technologies to address skill shortages effectively.
- Bridging the gap: Without the right skillsets, the cement industry’s growth and sustainability goals are at serious risk worldwide.
The urgent need to address the skill shortage
Time is running out. By 2024, the world will have a surplus of 1 billion tonnes of cement, but only skilled workers can make sure it’s used properly (World Cement Association, 2024). In India, the demand for cement could grow by 7–8 per cent every year through 2027, needing $14.89 billion in new plants (CRISIL, 2024). But without the right skills, this growth is at risk.
Here’s the hard truth for cement companies: current efforts aren’t enough. Training 10,000 workers, like UltraTech did, is a good start, but India needs 500,000 more skilled workers by 2030 to keep up with demand. Globally, companies are hoarding technology but aren’t sharing the necessary training, leaving developing regions behind. In India, companies often focus more on profits than on developing talent. Southern plants, for example, lower wages instead of investing in training, causing skilled workers to leave for better-paying jobs in IT. As for sustainability? Many green projects are just for show unless workers have the skills to run them.
So, what’s the solution? Companies need to think bigger. Globally, the cement industry should pool resources and create an industry fund for training, like the oil industry does for research and development. They should share training tools, like virtual reality, to help regions like Africa catch up. In India, companies like UltraTech and Adani should take the lead by partnering with all engineering colleges—not just the top ones—and offering scholarships to attract young talent. Rural plants should raise wages to compete with city jobs. Governments can help too, like India’s 2025 budget could offer tax cuts to companies that train workers, similar to Germany’s model.
But here’s the tough reality: many cement companies resist change. They prefer to squeeze more out of their current workforce rather than investing in new talent. That approach won’t work. If they don’t act now—really act—plants will shut down, projects will be delayed, and sustainability goals will fail. The choice is clear: build a skilled future or watch everything collapse. Which path will you take?
Conclusion
The skill shortage in cement manufacturing is a serious issue, both globally and in India, and it can’t be ignored any longer. As older workers retire, technology is taking over, but young people aren’t interested in cement jobs. This is causing growth to slow down and impacting green initiatives worldwide. In India, with its massive cement production of 410 million tonnes a year and big plans for the future, the situation is even more critical—65 per cent of plants lack skilled workers, putting jobs and infrastructure at risk.
However, there’s hope. New training programmes, like UltraTech’s centres or global VR training tools, show progress, but these efforts aren’t enough yet. Companies must act quickly, share ideas and invest more in training their workforce. If India makes the right moves, it could boost its production to 599.7 million tonnes by 2032 (IMARC Group, 2024). The global cement industry can also thrive with the right investment in skills.
Ignoring the skill gap means risking the foundation of the cement industry—and the future of construction worldwide. Cement companies must decide now: invest in skilled workers and grow, or let the industry’s progress crumble.
References
1. Confederation of Indian Industry (CII). (2024). Skill shortages in Indian manufacturing: A sector-wise analysis. New Delhi: CII.
2. CRISIL. (2024). Cement sector to invest US$ 14.89 billion as capex by FY27. Mumbai: CRISIL Ratings.
3. Fortune Business Insights. (2025). Cement market size, share & trends: Growth report [2032].
4. India Brand Equity Foundation (IBEF). (2024). Indian cement industry analysis.
5. International Energy Agency (IEA). (2023). Cement: Reducing CO2 emissions while meeting demand.
6. IMARC Group. (2024). India cement market size, share, demand & growth – 2032.
7. McKinsey & Company. (2023). The future of manufacturing: Talent trends in 2023.
8. National Skill Development Corporation (NSDC). (2024). Skill gap assessment in the Indian cement sector. New Delhi: NSDC.
9. Rhodium Group. (2024). The global cement challenge.
10. Statista. (2024a). India’s cement production volume 2008–2022.
11. Statista. (2024b). China’s cement production volume 2010–2023.
12. Statista. (2025). Cement production global 2023.
13. US Geological Survey. (2024). Major countries in worldwide cement production in 2023.
14. World Cement Association. (2024). Global cement industry outlook: Trends and forecasts.
15. World Cement. (2025). India in focus: A comprehensive analysis of the Indian cement industry.
About the author:
Dr SB Hegde is a Professor with the Department of Civil Engineering, Jain College of Engineering and Technology, Hubli, India and a Visiting Professor at Pennsylvania State University, USA. He is a globally recognised cement industry expert and academic leader with over three decades of experience. He has held senior positions in leading cement companies and has authored over 235 research papers, holds 10 patents and guided 2 PhDs.
Concrete
Solving AF Processing Challenge with Advanced Combustion
Published
19 hours agoon
April 18, 2025By
Roshna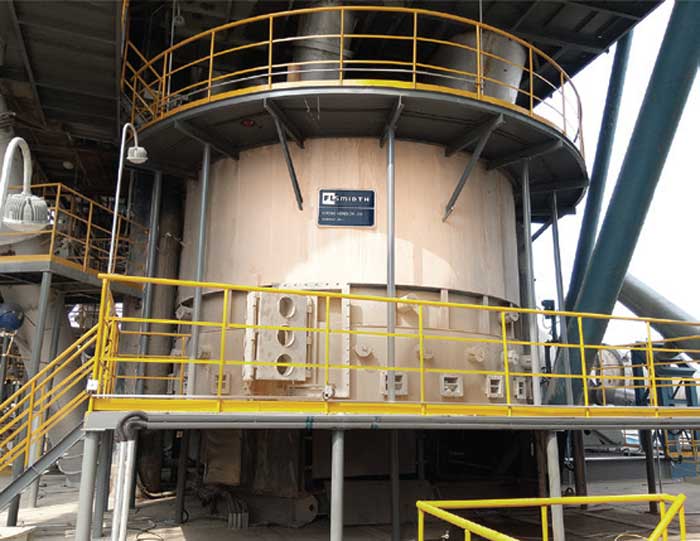
Alternative fuels reduce cement plants’ carbon footprint, but infrastructure challenges limit adoption. Technologies like the HOTDISC® Reactor help overcome these barriers.
Alternative fuels are a relatively straightforward and readily available means of reducing a cement plant’s carbon footprint. The technology is proven and well used worldwide, and with the right controls in place the switch from fossil fuels to waste-derived fuels does not impact the quality of the end product. In some countries, cement plants are achieving near 100 per cent substitution in the calciner and high levels of substitution in the kiln. However, this trend is not universal, and some countries are struggling to achieve a thermal substitution rate (TSR) of 25 per cent. In this article, we will look at the obstacles to alternative fuels use and the technology that is available to overcome them.
Advantages of alternative fuels
Alternative fuels offer three key environmental advantages.
1) A lower carbon alternative to coal or petcoke.
2) A pathway for waste that might otherwise be landfilled, including hazardous waste.
3) An alternative to waste incineration, which is typically done at lower temperatures where emissions tend to be higher.
In addition, the cost of alternative fuels can often be lower than fossil fuels and is not subject to the fluctuations of the energy market.
The sources of alternative fuels are many and varied – to the extent that the supply chain looks vastly different from one region to the next. For example, whereas India has abundant sources of biomass such as rice husk, in Western Europe there are plentiful supplies of refuse-derived fuel (RDF). This is partly a matter of industry and partly of infrastructure. But given the importance of reducing the cement industry’s reliance on coal, a lack of infrastructure must not prevent greater utilisation of alternative fuels – which is why FLSmidth Cement has for some time been developing alternative fuels solutions that reduce the burden of pre-processing and enable cement plants to more easily and more cost-effectively utilise a wide variety of waste streams.
A solution for all waste
Direct calciner injection may seem like the simplest way to replace fossil fuels with alternatives. However, it’s not always the best. The options for alternative fuels are limited by the necessity to pre-process fuel in preparation for burning, which, as stated, requires established infrastructure, or additional facilities at the plant.
Though the CAPEX cost of direct calciner injection is low, the calciner fuel substitution rate is also low, so this method doesn’t enable cement plants to optimise the potential for fuel replacement. Plant operators must also consider the impact on the process of direct injection, which doesn’t allow the long residence time that can be required to reduce process volatility. No plant wants to contend with greater instability or an increase in emissions from adding alternative fuels to the mix. Fortunately, direct calciner injection is not the only option. There are other ways of extracting energy from waste that require no pre-processing at all.
The HOTDISC® Reactor can handle a wide variety of solid waste in sizes up to 1.2m – from sludge or grains to whole truck tyres. There’s no need for expensive shredding or pre-drying, or any pre-processing, which removes one of the obstacles to adopting alternative fuels. The broad range of accepted fuel types also means cement plants are free to shop the market and not tied into one supplier. This makes it a very cost-effective solution because cement plants can select the lowest cost fuel without worrying about the quality.
How does the HOTDISC® work?
The HOTDISC® is a moving hearth furnace that is integrated into the pyroprocess below the calciner bottom and above the kiln riser. Coarse alternative fuels are fed onto a slowly rotating disc. Hot tertiary air is directed into the HOTDISC to provide an oxidising atmosphere for the alternative fuel to burn. As the alternative fuel slowly travels around approximately 270 degrees on the rotating disc, almost all of it fully combusts. Depending on the nature of the alternative fuel (size, heat content, moisture, etc.), the rotational speed of the HOTDISC can be adjusted to optimise the residence time (up to 45 minutes) and combustion rate. In addition, the temperature inside the HOTDISC is controlled by directing a portion of the preheated raw meal into the HOTDISC. The HOTDISC operation generates a controlled mix of hot gases, combustion residue (ash) and calcined raw meal that exits the HOTDISC. The combustion gases and finer materials are carried with the hot gases into the bottom of the calciner, while the coarser residues meet a scraper at the end of the 270 degrees rotation, where they are directed down into the riser duct. From there, this material falls into the kiln and is incorporated into the clinker.
The HOTDISC is designed to achieve a calciner substitution rate in the range of 50 to 80 per cent – or even higher – of the calciner fuel. Results vary by the specific plant conditions and fuel specification, but based on over 20 years of plant data it is possible to predict the substitution rate in each application.
The HOTDISC was originally designed for use with In-Line Calciners (ILCs), but new models are now available for use with Separate Line Calciners (SLCs), enabling the HOTDISC to be installed under the calciner and still deliver the same benefits. The HOTDISC-S is installed in the bottom part of the SLC calciner on the ground, the reject will be cooled and transported to a container or back into the system, gas flow and AF flow operates counter current.
For cement plants that wanted to utilise a wide range of alternative fuels, the HOTDISC®-S is a cost-saving solution that avoids the expense of changing the SLC to an ILC while enabling a high substitution of alternative fuels. Another model, the HOTDISC®-HMT (Hot Material Transport), enables quicker and easier installation of the HOTDISC in existing plants. Instead of directly integrating the exit of the HOTDISC reactor to the calciner and riser duct, the new layout allows the HOTDISC reactor to be mounted two to five metres away. It is then connected to the calciner and riser duct via a hot material transport chute for gas flows and combustion ashes.
Further advances in alternative fuels technologies
Low or varying quality alternative fuels can be another inhibitor to substitution, given the requirements of the relatively delicate cement pyro process. FLSmidth Cement has expended considerable R&D effort developing solutions that can accommodate a wide range of fuel types, knowing that this is the easiest path to greater substitution and ultimately the near-elimination of fossil fuels. The FUELFLEX® Pyrolyzer was one result of this effort and offers an exciting prospect for cement plants wishing to achieve near – 100 per cent substitution in the calciner and minimise NOx emissions.
The FUELFLEX® Pyrolyzer utilises hot meal from the lower preheater cyclones (yellow arrows) to dry and pyrolyze RDF or biomass. Either part or the full stream of hot meal from a lower preheater cyclone is admitted to the Pyrolyzer via the U-Lock (controlled by two dividing gates). The U-Lock fluidises the hot meal, forming a U-shaped gas lock that prevents pyrolysis gases from flowing backwards through the process. Subsequently the hot meal stream flows into the Pyrolyzer vessel, which also has a U-shaped lower aerated section to contain the hot fluidised meal. Fuel is pneumatically fed to the pyrolyzer vessel wherein through contact with the hot meal, it is dried, heated and pyrolyzed to form reactive gases and char. The gases push upwards into the main pyrolyzer vessels while the char falls down into the fluidised meal bed, before being reunited and fed as a very reactive stream into the calciner. Aeration panels are used to fluidise the hot meal and drain gates are used to drain out debris and meal from the pyrolyzer to the kiln system in a controlled manner. The reactive stream of pyrolysis products reacts with rotary kiln NO by so-called ‘re-burning’ reactions, utilising pyrolysis gases to convert NO into free N2 in the reduction zone prior to mixing with preheated combustion air in the calciner. In addition, the full fuel pyrolysis preceding the calciner helps limit calciner NOx formation by limiting access to oxygen when burning.
By using the FUELFLEX® Pyrolyzer, cement plants can achieve up to 100 per cent fossil fuel replacement in the calciner, with the following benefits:
- Reduced CO2 emissions, as net CO2 emissions from alternative fuels generally are lower than from fossil fuels.
- Increased utilisation of local waste streams, avoiding the need to dispose of or store this waste in other ways.
- Reduced fuel costs, especially in times of fluctuating energy prices.
- Reduced fossil fuel use saves the associated environmental impact of fossil fuel extraction and transport.
Conclusion
The challenge is on: cement plants must reduce carbon emissions now, and continue to do so for the next several decades until the target of net zero is met. While there are some solutions that are not ready yet – i.e. carbon capture – alternative fuels offer a valuable means of reducing the cement industry’s environmental impact immediately, with the added benefit of providing a controlled means of waste disposal. New and proven technologies will help the cement industry to overcome alternative fuel supply chain problems and achieve a dramatic reduction in fossil fuel use.
(Communication by the management of the company)

Concrete Through the Lens

Bridging the Skill Gap in Manufacturing

Solving AF Processing Challenge with Advanced Combustion

We consistently push the boundaries of technology

Women in Home Ownership

Concrete Through the Lens

Bridging the Skill Gap in Manufacturing

Solving AF Processing Challenge with Advanced Combustion

We consistently push the boundaries of technology

Women in Home Ownership
Trending News
-
Concrete4 weeks ago
Driving Sustainability Through Innovation
-
Concrete1 month ago
Transforming Interior Spaces: Trendy Wall Putty Designs to Enhance Your Home
-
Economy & Market4 weeks ago
Showcasing India’s Supply Chain Revolution
-
Economy & Market4 weeks ago
Highlighting the Future of Smart, Sustainable Infra