Environment
Optimising Energy Efficiency
Published
10 years agoon
By
admin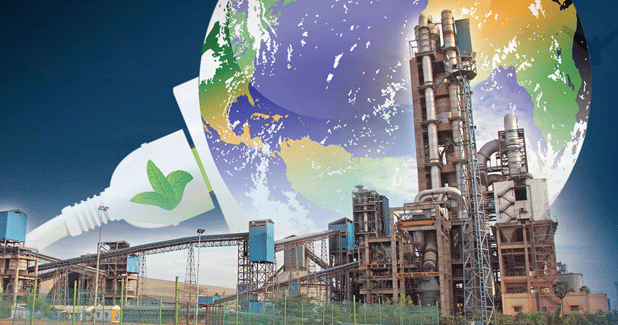
The efforts of Indian cement industry moving towards greater sustainability are getting strengthened by the use of increasing clinker substitution, use of alternative fuels and installation of WHR systems.
Energy efficient cement plants in the country are comparable to the best cement plants in the world. In India, these modern plants co-exist with older plants with lower capacities and obsolete technologies, and the poor performance of some of the plants brings down the average. The average electrical energy consumption in India is around 97 kWh/tonne of cement (OPC) whereas the best achievement is around 77 kWh/tonne of cement (OPC). Similarly, average thermal energy consumption is around 770 kcal/kg of clinker, whereas the best figures are around 680 kcal/kg of clinker.
Challenges
Even in this era of consolidation and acquisitions, the major challenge that dents the healthy growth of the cement industry is the sheer lack of demand; the realty and housing sector which consumes over 67 per cent of the total production is still not out of woods, nor there is a demand pull from the infra sector, as has been expected. The average capacity utilisation is around 70-72 per cent of the total installed capacity. This adversely impacts the energy efficiency drive by the cement manufacturers as running the plants at 70-80 per cent of installed capacity will drive out any of the benefits perceived in installing good equipment or carrying out energy management drives.
Sustained energy efficiency is possible only when plants run continuously with hundred per cent runtime and greater than hundred per cent production factor. Says Jagdish Chandra Toshniwal, Executive Director, Wonder Cement, ?It is absolutely right that the plant runs efficiently when it is operating at its full capacity. Continuous running of a plant is extremely important for energy efficiency as well as for lower maintenance cost. The inefficiency creeps in when it runs below certain optimum level and every plant knows, what its optimum level is. To have lowest cost of operation it is ideal to run the plant between 90 to 105 per cent capacity. Beyond 105 per cent capacity utilisation, the plant starts consuming more power and fuel. Where as anything lower than 90 per cent, your operational cost increases. When we are constrained to operate below 90 per cent, may be because of market conditions, we have to compromise on energy efficiency parameters. Also it is important to note that the maintenance cost is the lowest when we run the plant; under slightly stretched condition, say 105 per cent capacity.?
Says Dr. Suchismita Bhattacharya, Head – Process, Penta India Cement & Minerals, ?State of the art dry process cement plants are designed for specific energy consumption of around 697 kcal/kg clinker and around 90 kWh/tonne of OPC within the boundry of the cement factory from raw material storage to cement despatch. Having said that, achieving such levels in regular operation depends on various factors such as run time and capacity utilisation. Hence it is important to maintain at least 95 per cent of design production levels to take advantages of the new technologies. In some cases, use of variable frequency drives in certain equipment such as process fans may allow one to operate efficiently even at lower capacity levels. In the last few years, as the plants have been running at 60-70 per cent of installed capacity, higher operating costs per unit of production have been observed.?
Says Prashant K Tripathy, Executive Director – Productions & Processes, Dalmia Cement Bharat Ltd, ?Frequent stoppages and restart causes loss of power and fuel, both. Currently the cement demand is sluggish and the industry is awaiting a pickup in cement consumption to operate the plants efficiently at full capacity.?
Advantage AFR
Another major challenge from the energy efficiency front is the low thermal substitution rate by use of alternative fuels and raw materials (AFR). Even though the Indian cement industry has made a significant improvement in energy efficiency through various measures, use of AFR, a major potential area for improvement, is still in its nascent stage; the present thermal substitution rate by use of AFR being in the range of 0.5 to 1 per cent whereas developed countries achieved as high as 40 per cent TSR.
Says KN Rao, Director, Environment and Energy Conservation, ACC Ltd, ?ACC has been using successfully close to half a million tonnes of alternative fuels and raw materials annually and hope to increase substantially this quantity in the coming years as necessary infrastructure has been created in most of the plants. This initiative is helping the entire country for safe and sustainable disposal of the hazardous waste and non hazardous waste of various industries and municipal solid waste and helping the country to achieve energy security and minimising the GHG footprint of the country.?
Dr. Suchismita adds, ?Alternate fuels and refused derived fuels have considerable scope in India. It is possible to reduce the gate to gate energy consumption of renewable fuels by substituting with such fuels. In India some of the plant owners have achieved 10-15 per cent substitution. However a certain amount of capital investment in fuel handling facilities within the cement plant, fuel firing technology,selection of burner, calciner design, refractory selection and laboratory automation may be warranted. Dealing with the varying nature of the fuel will require expert adjustments in fuel mix. Another hurdle in India is that waste derived fuel is not available from Municipalities in an organised manner. However as Europe has shown the way, over 80 per cent substitution is possible all the variables can be dealt with as long as the management shows the will to use alternate fuel.?
Says Toshniwal, ?In fact the time is ripe to start initiating action in that direction. The leading companies like ACC and Ambuja are the torch bearers. We shall follow them. On the role of regulators, it is changing very fast but there seems to be some confusion. For getting approval we have to go through several layers. The agencies involved are CPCB and State Pollution Board. Sometimes they are not clear as to who will approve what? The procedures have been simplified because even the state officials are realising that waste disposal is a great problem today. There is a positive side, as the mind set of government officials, pollution control board is changing.? Avers Tripathy, ?Assured long term availability of AFR to justify infrastructure related capex is the main challenge. Requirements of environment permits for each new AFR is a major issue. For small volumes the cost of trials needed is not justified. Land filling is still the preferred option for a large volume of hazardous waste and municipal solid waste.No economic model is available to derive cement quality RDF from MSW for use in cement plants as AFR.?
Optimising clinker content
The cement industry being a highly energy-intensive sector, most of the major players have been adopting the best manufacturing practices right from mining to production to sales and distribution, across all units and disciplines by optimising energy, natural resources and technology. The Indian cement industry, over the years, has employed the best available technology for production; thanks to a high degree of blended cement utilisation, Indian cement producers are at the forefront of fuel and electrical energy consumption on a per tonne- of- product basis. An additional benefit in terms of sustainability is the lower per tonne CO2 emission. Stricter regulatory requirements are leading to greener technologies, and they in turn, lead to further energy efficiency.
From the energy efficiency perspective, consistent availability of quality fly ash is another constraint as it impacts optimisation of clinker component per tonne of cement produced by using fly ash. According to Rao, there is a greater scope of using fly ash and other cementitious materials in cement manufacturing by actively promoting blended cements and composite cements. Present Indian Standards for PPC allows 35 per cent absorption of flyash in cement manufacturing process. Similarly Indian Standards for Portland Slag Cement (PSC) which allows 65 per cent absorption of slag in cement manufacturing process. Composite cement standards which are currently under draft stage are expected to permit higher usage of pozzolona materials in cement manufacturing. The above initiatives will result in lower consumption of clinker in cement manufacturing. Since the clinker manufacturing involves huge amount of thermal energy, electrical energy consumption and CO2 emissions, any reduction in the clinker content in cement will reduce specific thermal energy, electrical energy and CO2 emission in cement manufacturing.
Says Rao, ?The cement industry is the biggest consumer of fly ash in India and ACC is the leading manufacturer of blended cements. ACC is consuming 4.4 million tonne of fly ash and 2.8 million tonne slag annually for production of blended cement. Use of slag and fly ash in cement manufacture helps the steel industry and power plants to dispose of their waste in an environment friendly manner while reducing the GHG emission from the cement industry and also minimising the use of limited resources like limestone.?
Low-carbon technology
Following the global Cement Technology Roadmap in 2009 developed in a partnership between WBCSD CSI and the International Energy Agency (IEA), nine CSI members in India again joined hands with IEA to develop a Technology Roadmap: Low-Carbon Technology for the Indian Cement Industry. The initiative in India is supported by the International Finance Corporation (IFC), a member of the World Bank Group. The India roadmap, launched in February 2013, outlines a low-carbon growth pathway for the Indian cement industry that could lead to carbon intensity reductions of 45 per cent by 2050. It proposes that these reductions could come from increased clinker substitution and alternative fuel use; further improvements to energy efficiency, and the development and widespread implementation of newer technologies.
Says Rao, ?We have developed many products which have a low carbon footprint and energy foot print aligning with our sustainable development road map. Our blended cement CDM project is the biggest CDM project in the cement sector in the country with annual CO2 emission reduction potential of approximately of 200,000 tonne of CO2/year.?
Says Prashant, ?In all our units we have taken the initiative to maximise the use of AFR as it is a key lever to reduce CO2 emissions. We are proud member of Cement Sustainability Initiative (CSI) and are tackling all challenges to use AFR as per CSI guidelines/charter.?
Waste heat recovery
According to Sunil Kumar Singh, Director – Metals, Mining & Minerals, Schneider Electric India, even though the investment is high for WHR applications, it is the major energy efficiency project on thermal side. He says, ?Power generation using WHR results in considerable savings and also Indian cement industry can take the advantage under PAT cycle.?
Speaking about the scope of WHR Dr. Suchismita had this to say. ?WHR projects were adopted by Indian owners in a big way during the last four years. However there are mixed experiences regarding the successful implementation of these projects. This is dissuading some of the owners who were on the fence from going ahead and finalising their planned WHR projects. In some of the WHRS projects, the process design basis was not selected properly and as a result, there were over promise of power generation. Moreover, equipment selection has to be made properly and workmanship has to be perfect when it comes to boiler tubes, etc. Steam Rankine cycle based power generation is a fairly mature technology and the technology providers have suited their designs for the exhausted gas of the cement plants. Newer technologies based on the Organic Rankine cycle and the Ammonia-based Kalina cycle may take a while to pick up as they are the more costly options. Says Rao, ?Typically, clinkerisation lines, in all cement plants, have a potential to generate power by utilising the waste heat from pre-heater and cooler gases using low pressure boilers and turbine. A 7.5 MW WHR system has been installed at our Gagal plant in Himachal Pradesh in 2014. We have plans to implement similar projects in other cement plant of ACC.? Speaking about the challenges of installing WHR system, Chander K, Technology Manager, India Operations Center, Process Automation, ABB India, says, ?High initial capital investment is almost always a deterrent to employing WHR systems. However, the benefits realised over a longer term in terms of utilising waste heat for generating power will pay off the high initial costs.?
Plant & equipment
The amount of energy saving varies on a case-to-case basis depending on the actual selection of process and equipment, quality and consistency of fuel, raw material characteristics, etc. However, it is important to understand here that long-term plant energy efficiency cannot be guaranteed based on the mere selection of the most efficient individual equipment alone. Rather, over a long term, energy (fuel and power) efficiency is largely driven by uniformity of the kiln feed chemistry, mastery of the burning zone which is primarily a kiln operation, and plant reliability factor i.e, avoiding stoppages due to incidents; all this in turn, relates to plant preventive maintenance.
Says Chander, ?The energy efficiency of cement production strongly depends on the process used to produce the clinker, the main component in cement manufacturing and the type of kiln. Dry kilns with pre-heaters and pre-calciners are the most efficient. The most efficient technologies used to produce cement are found in Japan, Mexico and in European countries, whereas the technologies used in Asian and North American countries are less efficient. Pre-heater, kiln and cooler section, raw mill, cement mill and coal mill are areas where tremendous scope is available in terms of energy savings.?
According to Singh on electrical side most of the cement plants have installed updated technology and process equipments. It is mainly grinding mill circuits, process fans and material conveying method which will determine the energy efficiency of the plant. Singh explains, ?The plants are considered to be energy efficient if they adopt the following:
Using vertical roller mills with full capacity utilisation and a high efficiency separator, ensuring minimum air ingress and pressure drop in circuit
Using process fans of high operating efficiency and using drives for flow control
Using mechanical conveying for material transport.
Utilising a vertical roller mill or roll press circuit in finish grinding mode for raw material grinding is the industry norm today, and this makes for a significant energy cost reduction when compared to the traditional closed circuit ball mill system. Likewise, for coal grinding, a vertical mill is used, and for the energy- intensive finish grinding process, the ball mill plus roll press system is widely popular. In specific cases where slag grinding is involved with high per centage moisture, the VRM technology for finish grinding is used. High efficiency separators are the standard today for all milling systems.
As regards the pyro-processing area, Indian cement producers continuously strive to achieve the lowest specific fuel consumption along with high power savings. High efficiency fourth- generation grate coolers are being widely used; they provide high recuperation efficiency along with lower maintenance interventions. As the total cooling air requirement reduces from the earlier 2.2 Nm3/kg clinker to say, 1.8 Nm3/kg clinker, there is a lot of savings through reduced exhaust air and fans` power consumption. To achieve lower fuel consumption, six stage pre-heater systems are the popular choice, along with in- line calciners. Advanced low NOx technologies are used in many cement plants. As regards process fans, a static efficiency = 82 per cent and use of variable speed drives reduces power consumption.
Towards sustainability
Sustainability awareness has picked up momentum in recent years in the cement industry, and several efforts are on by both cement manufacturers and major plant and machinery and auxiliary equipment manufacturers to integrate sustainability issues, essentially in energy conservation, resource optimisation and environmental planning, with business plans and reviews. For the cement industry, the major focus areas for sustainability are improving thermal energy efficiency and process technology, optimising fuel composition, including the use of waste as fuel, waste heat recovery, reduction in clinker factor, especially through increased rates of blending, and renewable energy. It is heartening to note that most of the cement companies have developed specific initiatives and road maps to reduce their organisational carbon footprint.
AGITH G ANTONY
ENERGY SAVING MEASURES
- Reduction of pressure drop of preheater tower
- Proper selection and operation of cooler,
- Improve the burner and operation.
- Improve raw mix design and its burnability
- Reduce raw material feed size especially for ball mill by close circuiting of crusher/installation of pre-grinder
- Improvement in plant productivity
- Reduce compressed air consumption and improve the pipe line routing.
- Improve fan efficiency: Low operating efficiency of fan is mainly due to wrong selection of fan type and its operating point being far off from the design point.
- Reduction in leakages across the system
- Reduction of the downtime/breakdown of the machine by proper maintenance.
- Improve the layout of the plant
- Any other case specific solution
Courtesy: Penta India
CASE STUDY: ABB
We received the first major order in the energy efficiency domain from Holcim in India, to improve specific power consumption of their cement plants. The solution offered included ACS 5000 and ACS 2000 range of 6.6 kV medium voltage (MV) drives that enhance energy efficiency at three plants, replacing existing damper controls. There is a huge potential to further enhance the efficiency by providing the MV drives for other process critical applications like preheater fans, mill separator fans, cooler fans in cement production area and also extend it to captive power plants to cover boiler feed pump (BFP), primary air and secondary air (PA/SA) fans and induced draft (ID) fans. Fans in the cement industry are usually large and consume a major part of electrical energy. Traditionally, Indian cement producers use cascade converters ? also called Slip Power Recovery Systems (SPRS) – to control the speed of process fans. However, due to inherent limitations of SPRS, such as operation in weak networks and higher harmonics, they are now considering the variable speed drive (VSD) solution. With the use of VSDs, the energy consumption can be reduced from 90 kWHs/tonne to about 70 kWHs/tonne of cement produced.
– Chander K, Technology Manager, India Operations Center, Process Automation, ABB India
You may like
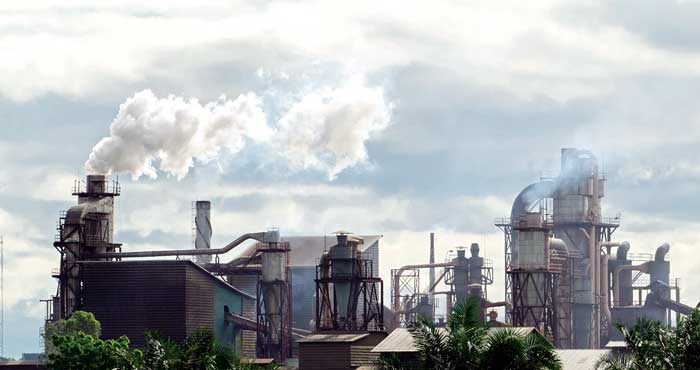
ICR discusses India’s rapid advances in renewable energy, on track to exceed its 2030 targets, even as the rising energy demands challenge complete reliance on sustainable sources.
The cement industry, a cornerstone of infrastructure development, has long been associated with high emissions, particularly of CO2. This sector alone is responsible for approximately 8 per cent of global carbon dioxide emissions, primarily due to the energy-intensive processes of clinker production and calcination. Beyond carbon emissions, cement production also generates particulates, nitrogen oxides (NOx), sulphur oxides (SOx), and other pollutants, contributing to environmental degradation and health risks. With the global push towards sustainable practices and carbon neutrality, addressing emissions in the cement industry has become imperative.
According to Climate Change Performance Index, India ranks 7 in 2024. India receives a high ranking in the GHG Emissions and Energy Use categories, but a medium in Climate Policy and Renewable Energy, as in the previous year. While India is the world’s most populous country, it has relatively low per capita emissions. Data shows that in the per capita GHG category, the country is on track to meet a benchmark of well below 2°C.
India’s situation underscores the complexity of transitioning to sustainable energy systems in the face of rising and fluctuating energy needs. International support is crucial for India to access advanced technologies, financial resources, and best practices that can accelerate its transition to a sustainable energy future. Our analysis shows that with current policies, India will overachieve its conditional NDC targets of achieving 50 per cent non-fossil capacity by 2030, so it could set stronger targets. India has ambitious renewable energy plans as outlined in the National Electricity Plan 2023 (NEP2023) aiming for a share of installed capacity of 57 per cent and 66 per cent in 2026-27 and 2031-32, respectively. Share of renewable energy capacity in India reached 44 per cent, ranked fourth in the world in renewable energy capacity installations in 2023, after China, the US and Germany. The NEP2023 is reflected in the lower bound of our current policy and action pathway.
India has seen a steady increase in renewable energy deployment, including both utility-scale and rooftop solar, leading to the share of coal capacity dropping below 50 per cent for the first time. However, this increase in renewable energy capacity is barely able to keep up with the surging demand. As a result, the electricity generation share of renewable energy, including large hydro, remains at around 18 per cent, showing no improvement since last year. Investment in renewable energy projects in India are projected to increase by over 83 per cent to around USD 16.5 bn in 2024, with fossil fuel companies also diversifying their investments into the renewable sector. Despite this, India has not committed to phasing out coal power or fossil gas.
The National Electricity Plan indicated a temporary halt in coal capacity addition, but current under-construction capacity exceeds the threshold stated in these plans. While new gas power projects have been abandoned, the utilisation of existing gas power plants has increased to meet energy demand driven by severe heat stress.
Understanding Emissions in Cement Production
Primary Sources of Emissions: Cement production emissions stem mainly from three sources: calcination, fuel combustion, and electricity use. During calcination, limestone is heated to produce clinker, releasing CO2 as a by-product. This process alone accounts for roughly 60 per cent of emissions in cement manufacturing. The remaining emissions result from burning fossil fuels in kilns to achieve the high temperatures needed for calcination and from electricity consumption across production stages.
Raju Ramchandran, SVP Manufacturing (Cluster Head – Central), Nuvoco Vistas, says, “We consistently track air emissions from fuel combustion in our cement manufacturing and power generation operations. The burning of fossil fuels releases pollutants such as Oxides of Sulphur (SOx), Oxides of Nitrogen (NOx), and Particulate Matter (PM), which require stringent monitoring.”
“We ensure compliance with regulatory standards by using the Continuous Emission Monitoring System (CEMS) to monitor these emissions. For the FY 23-24, both our stack and fugitive emissions have stayed within the permissible limits set by Pollution Control Boards. Moreover, our ongoing monitoring of fugitive emissions ensures that we meet the prerequisite air quality standards,” he adds.
In addition to CO2, the cement industry releases various pollutants that pose risks to air quality and public health. These include particulate matter, NOx, and SOx, which can lead to respiratory and cardiovascular issues, acid rain, and ecosystem imbalances.
Governments worldwide are setting increasingly stringent regulations to curb industrial emissions. Standards such as the EU Emissions Trading System and India’s National Action Plan on Climate Change encourage cement manufacturers to adopt cleaner technologies. Many countries now impose limits on NOx, SOx and particulate emissions, with the aim of minimising the industry’s environmental impact.
Challenges in Reducing Emissions
High carbon intensity of cement production: Cement’s high carbon intensity largely stems from the chemical reactions involved in transforming limestone into clinker, making emissions difficult to reduce without altering core processes. Additionally, achieving the necessary kiln temperatures requires significant energy, often derived from coal or natural gas.
Operational limitations: Altering the traditional cement production process can compromise the quality and durability of the end product. Adapting existing production lines for lower emissions involves extensive R&D and technical trials to ensure the finished cement meets industry standards.
Financial constraints: The cost of implementing green technology is high, creating economic challenges, particularly for smaller cement manufacturers. Equipment upgrades, energy-efficient kilns, and carbon capture facilities require considerable investment, which many companies find difficult to justify without strong financial incentives.
Balancing market demands and environmental goals: With global infrastructure demands rising, the cement industry faces pressure to meet growing production needs while simultaneously working to reduce emissions. Balancing these competing demands requires innovation, efficient resource management, and support from stakeholders.
Technological Innovations for Emission Reduction
Alternative fuels and energy sources: One of the most effective ways to reduce emissions is by replacing fossil fuels with alternatives like waste-derived fuels, biomass, or biofuels. Some manufacturers are incorporating solar and wind energy to power auxiliary processes, further reducing reliance on traditional energy sources.
Sudhir Pathak, Head- Central Design & Engg (CDE), QA, Green Hydrogen, Hero Future Energies, says, “The cement industry is one of the largest consumers of grid power (Scope 2) and also a guzzler of in-process fossil CO2 (Scopem1) including process-based CO2 through limekilns. Decarbonisation can be achieved only up to 50 per cent to 60 per cent through plain hybrid solar and wind. However, for achieving balance 40 per cent, storage is essential, be it chemical or mechanical. Today, HFE is ready to provide such bespoke storage solutions as is evident through several complex RTC tenders that we have won in the last 6-8 months floated by agencies like SECI, NTPC and SJVN. These include tenders for FDRE projects, peak power, load following, etc. Further, regarding green hydrogen and its derivatives, we are ready to apply these for decarbonising industrial heating and mobility.”
Carbon Capture and Storage (CCS): CCS technology captures emissions at the source, storing CO2 to prevent it from entering the atmosphere. Recent advancements in CCS technology make it a viable option for large-scale cement plants, although high costs and infrastructure requirements remain obstacles to widespread adoption.
Clinker Substitution: Reducing clinker content is a promising method for emission reduction, achieved by using supplementary cementitious materials (SCMs) such as fly ash, slag, and calcined clay. These materials not only reduce CO2 emissions but also enhance the durability and performance of cement. SCMs are gradually becoming industry-standard components, especially in eco-friendly and green cement products.
Rajesh Kumar Nayma, Assistant General Manager – Environment, Wonder Cement, says, “The use of AFR plays a critical role in our strategy to reduce the environmental footprint of cement production. By substituting traditional fossil fuels with waste-derived alternatives like biomass, refuse-derived fuel (RDF) and industrial by-products, we significantly lower CO2 emissions and reduce the demand for natural resources. The utilisation of supplementary cementitious materials (SCMs), such as fly ash, helps in reducing clinker consumption, which is a major source of carbon emissions in cement production. This not only decreases our reliance on energy-intensive processes but also promotes waste recycling and resource efficiency. AFR adoption is an integral part of our commitment to the circular economy, ensuring that we minimise waste and optimise the use of materials throughout the production cycle, ultimately contributing to a more sustainable and eco-friendly cement industry.”
“WCL is exploring transitioning from fossil fuels to cleaner alternatives like biofuels or hydrogen or RDF/plastic waste/other hazardous waste. Till date, 5 per cent TSR has been achieved, while the intent is to achieve more than 20 per cent TSR. WCL is utilising the hazardous and other waste as an alternative fuel or raw material. We have used more than 3 lakh metric tonne of hydrogen waste and other waste in FY-2023-24,” he adds.
Improving energy efficiency is critical for emissions reduction. Technologies like high-efficiency kilns, heat recovery systems, and process optimisation techniques are helping manufacturers achieve more output with less energy. These measures reduce the carbon footprint while lowering operational costs.
The Role of SCMs
SCMs serve as partial replacements for clinker, providing a dual benefit of reduced carbon emissions and improved product resilience. The use of materials like fly ash and slag also helps mitigate industrial waste, contributing to a circular economy. Fly ash, slag, and silica fume are among the most widely used SCMs. Each has unique properties that contribute to cement’s strength, workability, and durability. By incorporating SCMs, manufacturers can produce cement with a lower environmental footprint without compromising quality.
While SCMs are effective, several obstacles hinder their widespread adoption. Supply chain constraints, material variability, and lack of technical standards are challenges that manufacturers face. Additionally, geographic limitations impact access to certain SCMs, creating disparities in their usage across regions.
Policy and Industry Collaboration
Policies play a critical role in driving green transitions within the cement industry. Carbon credits, tax incentives, and funding for R&D are some measures governments have introduced to support emission reduction. India’s Perform, Achieve, and Trade (PAT) scheme is an example of a policy incentivising industrial energy efficiency.
Collaborations between government entities, private corporations, and research institutions foster innovation and accelerate the adoption of sustainable practices. Partnerships can also help address funding gaps, allowing companies to explore new technologies without bearing the full financial burden.
International frameworks such as the Paris Agreement and industry-led efforts like the Global Cement and Concrete Association (GCCA) are setting targets for sustainable cement production. These initiatives encourage the sector to adopt environmentally friendly practices and set a roadmap toward achieving net-zero emissions.
Towards a Net-Zero Future
Reaching net-zero emissions is an ambitious but necessary goal for the cement industry. Realistic targets, set with interim milestones, allow companies to gradually transition to greener processes while maintaining production efficiency. Continued investment in R&D is crucial for discovering new methods of emission reduction. Emerging technologies such as carbon-negative materials, alternative binders, and low-carbon clinkers hold promise for the future, potentially transforming cement production into a more sustainable process.
Increasingly, consumers and investors are prioritising sustainability, placing pressure on companies to reduce their environmental impact. This shift in consumer sentiment is driving the cement industry to adopt green practices and focus on transparency in emissions reporting.
Conclusion
The journey toward reducing environmental impact in the cement industry is complex and multifaceted, requiring a combination of innovation, policy support, and industry collaboration. By adopting alternative fuels, implementing carbon capture technology, integrating SCMs, and improving energy efficiency, the industry can take significant strides in minimising its carbon footprint. Achieving sustainability in cement production is essential not only for the industry’s future but also for the planet’s well-being. Together, industry players, policymakers, and consumers can support the transition to a net-zero future, ensuring that cement remains a vital yet sustainable component of global infrastructure.
– Kanika Mathur
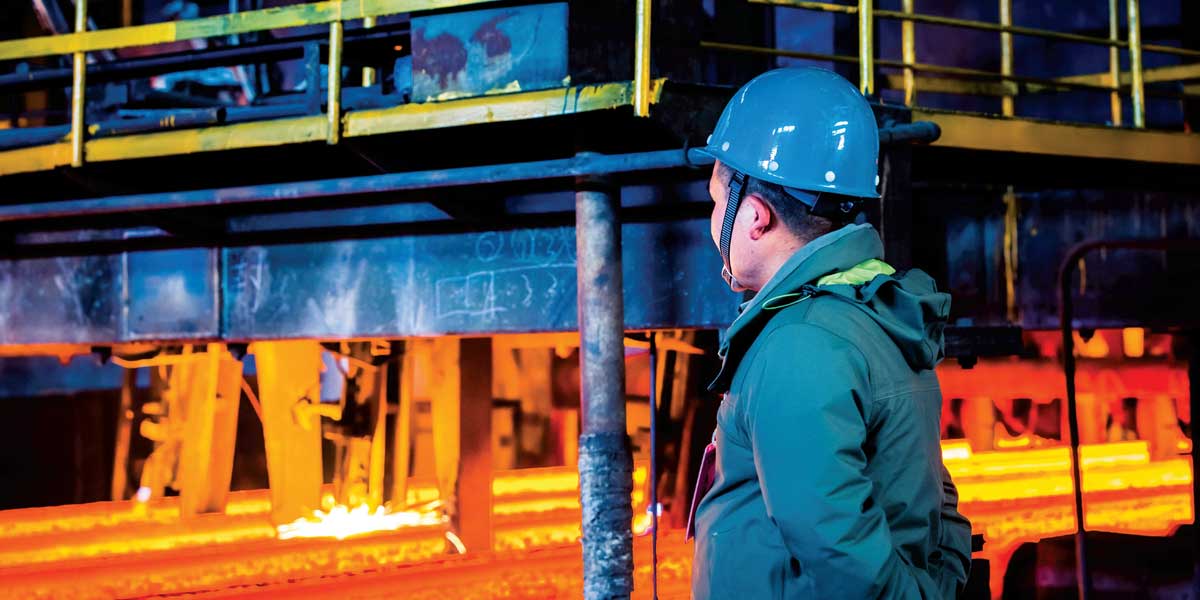
Dr SB Hegde, Professor, Jain College of Engineering and Technology, Hubli, and Visiting Professor, Pennsylvania State University, USA, helps us understand the red river formation in cement kiln operations, its causes, impacts and mitigation strategies.
Red river formation in cement kilns, where molten clinker flows uncontrollably in the cooler, is a costly problem for cement plants. The phenomenon not only affects clinker quality but also leads to significant operational disruptions, increased energy consumption and accelerated wear on kiln refractory bricks. Understanding the factors that cause red river formation and implementing strategies to prevent it are critical to maintaining operational efficiency and clinker quality.
This paper explores the causes of red river formation, the operational impacts it has on kiln performance, and the various mitigation strategies that cement plants can adopt. Additionally, safety considerations associated with the prevention and handling of red river formation are discussed, with practical insights from case studies of successful plant interventions in India and globally.
Causes of red river formation
Red river formation is primarily caused by improper kiln operations, including fluctuating kiln temperatures, oxygen levels, and cooler inefficiency. The following parameters are essential contributors:
Kiln temperature: Inconsistent temperature control in the kiln’s burning zone, often exceeding 1500°C, creates an imbalance between the solid and molten clinker phases, leading to red river formation. Maintaining temperatures within a more stable range of 1470-1490°C ensures that the clinker remains solid as it moves into the cooler.
Oxygen levels and CO concentrations: Oxygen levels above 2.5 per cent increase the risk of over-combustion, while elevated CO levels above 0.3 per cent indicate incomplete combustion, both contributing to excessive clinker melting. Optimising oxygen levels to 1.8-2.0 per cent minimises the risk.
Raw mix composition: The raw mix plays a vital role in clinker formation. A high liquid phase due to improper ratios of silica, alumina, and iron oxide can lead to excessive melting. Controlling the silica modulus (SM: 2.3-2.7) and alumina modulus (AM: 1.3-1.8) ensures a more stable clinker and reduces the risk of red river formation. If the raw mix is improperly proportioned, red river formation becomes more likely due to high fluxing compounds that melt at lower temperatures.
Kiln speed and torque: Kiln speeds that fluctuate below 3.4 rpm can cause material buildup, while kiln torque exceeding 50-60 per cent indicates stress that can lead to clinker instability.
Cooler efficiency: Inefficiencies in the clinker cooler, with efficiency levels below 78 per cent, can exacerbate red river formation. Clinker that is not cooled properly will remain molten for longer, allowing it to flow uncontrollably. Coolers should maintain exit temperatures between 180-200°C to prevent red river incidents.
Impact on clinker quality and kiln performance
The occurrence of red river has numerous negative impacts on both clinker quality and kiln performance:
Clinker quality: Red river formation results in poor clinker grindability, higher variability in free lime content and inconsistent cement properties. Poor clinker reactivity reduces both early and late strength development in the final cement product.
Increased heat consumption: Red river typically increases specific heat consumption by 3-5 per cent, resulting in higher fuel usage. These inefficiencies can significantly affect the plant’s cost structure, driving up operational expenses.
Refractory damage: The molten clinker accelerates the wear of refractory bricks in the kiln, especially in the burning zone and cooler transition areas. Brick life can decrease by 25-30 per cent, leading to more frequent replacements and higher maintenance costs.
Equipment and instrumentation damage: The uncontrolled molten flow of clinker during red river incidents can damage cooler plates, kiln discharge systems, and even temperature sensors and thermocouples, leading to costly repairs and prolonged downtime.
Mitigation strategies
Mitigating red river formation requires a multi-faceted approach combining operational optimisation, automation and staff training:
Kiln temperature control: Maintaining stable burning zone temperatures in the 1470-1490°C range is key to preventing excessive melting of clinker. Advanced temperature monitoring systems can help regulate temperature fluctuations.
Cooler efficiency optimisation: To ensure proper cooling, cooler efficiency must be maintained at 78-80 per cent, with clinker exit temperatures not exceeding 200°C. Real-time airflow adjustments in grate coolers improve cooling performance, solidifying the clinker at the appropriate stage.
Automation and data analytics: Advanced Process Control (APC) systems using data analytics can monitor critical kiln parameters—such as temperature, oxygen levels, and torque—in real-time, allowing for predictive maintenance and early intervention when red river signs appear. This technology has been implemented successfully in leading plants globally to prevent red river formation.
Indian case studies
Case Study 1: Cement Plant in South India – Optimisation of Kiln Parameters
A cement plant in South India faced recurrent red river issues due to high kiln temperatures and low cooler efficiency. After comprehensive process audits, the plant optimised its kiln temperature to 1480°C, reduced oxygen levels to 1.9 per cent, and upgraded its cooler to an efficiency of 80 per cent. These changes reduced red river incidents by 85 per cent, saving the plant Rs 10 million in energy costs annually and improving clinker quality by
15 per cent.
Case Study 2: Cement Plant in North India – Cooler Upgrade and Automation
A northern India plant increased cooler efficiency from 70 per cent to 78 per cent by installing an advanced grate cooler. This reduced clinker exit temperatures to 190°C, preventing red river formation. Automation systems provided real-time adjustments, decreasing the frequency of incidents by 75 per cent and saving `12 million annually.
Global Case Studies
Case Study 1: European Plant – Automation Success
A German cement plant, experiencing red river issues due to fluctuating oxygen levels, installed an advanced data-driven automation system. The system stabilised oxygen at 1.9 per cent and maintained kiln temperature at 1,475-1,485°C, reducing red river by 90 per cent. Clinker quality improved by 10 per cent, with a reduction in specific heat consumption by 4 per cent.
Case study 2: US Plant – Operator Training and Process Optimisation
A US cement plant reduced red river occurrences by 70 per cent through kiln speed optimisation (3.8 rpm) and comprehensive operator training. Improved monitoring of kiln torque and cooler exit temperatures led to higher cooler efficiency (75 per cent) and an annual savings of $2 million.
Safety Aspects
Safety is a paramount concern in red river incidents. When molten clinker flows uncontrollably, it poses a significant risk to personnel working near the kiln and cooler areas.
To mitigate these risks:
- Clearance zones: Kiln and cooler areas should have strict clearance zones for personnel when red river incidents are detected.
- Protective gear and training: Personnel should be equipped with proper protective equipment (PPEs) and trained to handle emergencies involving molten clinker. Emergency shutdown procedures should be well-documented and rehearsed.
- Automation and early warning systems: Automation can provide early warning systems that alert operators to potential red river formation before it becomes critical, ensuring safe intervention.
Conclusion
Red river formation remains a major operational challenge for cement plants, but it can be effectively mitigated through proper kiln temperature control, cooler efficiency optimisation and the use of advanced automation systems.
The case studies highlight the importance of process improvements and staff training in reducing red river occurrences, improving clinker quality, and lowering operational costs. Additionally, safety
measures must be prioritised to protect personnel from the risks posed by molten clinker. By incorporating these strategies, cement plants can ensure consistent kiln performance and enhanced operational efficiency.
References
1. Duda, W. H. (1985). Cement Data Book. International Process Engineering in the Cement Industry. Bauverlag GmbH.
2. Javed, I., & Sobolev, K. (2020). “Use of Automation in Modern Cement Plants.” Cement and Concrete Research, 130, 105967.
3. Tamilselvan, P., & Kumar, R. (2023). “Optimisation of Kiln and Cooler Systems in Indian Cement Plants.” Indian Cement Review, 34(7), 42-48.
4. Martin, L. (2019). “Case Studies of Red River Mitigation in European Cement Plants.” International Journal of Cement Production, 12(2), 63-78.
5. Schorr, H. (2021). “Advanced Process Control in Cement Manufacturing.” Cement International, 19(3), 30-37.
6. Singh, V. K., & Gupta, A. (2022). “Impact of Raw Mix on Clinker Formation and Kiln Operations.” Global Cement Magazine, 14(4), 22-29.
About the author: Dr SB Hegde brings over thirty years of leadership experience in the cement industry in India and internationally. He has published over 198 research papers and holds six patents, with four more filed in the USA in 2023. His advisory roles extend to multinational cement companies globally and a governmental Think Tank, contributing to research and policy. Recognised for his contributions, he received the ‘Global Visionary Award’ in 2020 from the Gujarat Chambers of Commerce and Industry.
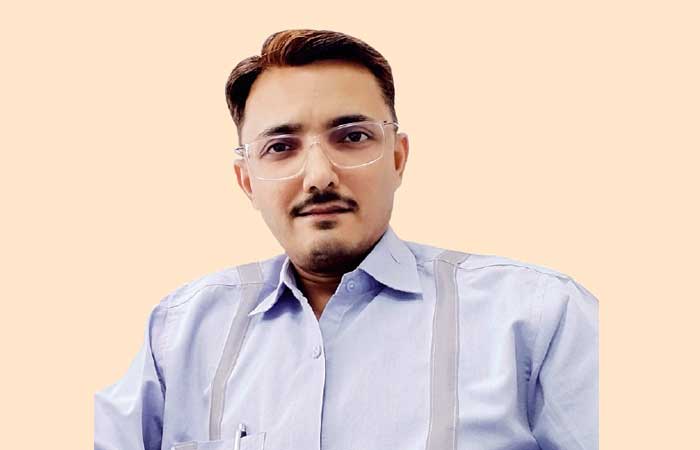
As the cement industry prioritises sustainability and performance, Supplementary Cementitious Materials (SCMs) are redefining standards, explains Tushar Khandhadia, General Manager – Production, Udaipur Cement Works.
What role do supplementary cementitious materials (SCMs) play in enhancing the performance and sustainability of cement and concrete?
SCMs play a crucial role in enhancing the performance and sustainability of cement and concrete. These materials are added to concrete to improve its properties such as strength, durability, and workability, as well as to reduce the environmental impact of cement production. The addition of SCMs to cement reduces the amount of Portland cement required to manufacture concrete, reducing the carbon footprint of the concrete. These materials are often industrial waste products or by-products that can be used as a replacement for cement, such as fly ash, slag and silica fume.
SCMs also reduce the amount of water required to produce concrete, which reduces the environmental impact of concrete production. This is achieved through their ability to improve the workability of concrete, allowing the same amount of work to be done with less water.
In addition, SCMs improve the durability of concrete by reducing the risk of cracking and improving resistance to chemical attack and other forms of degradation.
How has your company integrated SCMs into its production process, and what challenges have you encountered?
The integration of SCMs into cement and concrete production may pose certain challenges in the areas of sourcing, handling and production optimisation.
- Sourcing: Finding an adequate and reliable supply of SCMs can be a challenge. Some SCMs, such as fly ash and slag, are readily available by-products of other industrial processes, while others such as silica fume or metakaolin may be more difficult to source.
- Handling: The storage, handling, and transportation of SCMs require special considerations due to their physical and chemical properties. For instance, some SCMs are stored in moist conditions to prevent them from drying out and becoming airborne, which could pose a safety risk to workers.
- Production optimisation: The addition of SCMs into the mix may require adjustments to the production process to achieve the desired properties of cement and concrete. For example, the use of SCMs may affect the setting time, workability, strength gain, and other properties of the final product, which may require reconfiguration of the production process.
- Quality control: The addition of SCMs may introduce variability in the properties of cement and concrete, and rigorous quality control measures are necessary to ensure the final product meets the required specifications and standards.
Proper planning, handling and production optimisation are essential in overcoming the challenges encountered during the integration process.
Can you share insights on how SCMs such as fly ash, slag and silica fume impact the durability and strength of concrete in different environmental conditions?
- Fly ash is a by-product of coal combustion and is widely used as an SCM in the production of concrete. When added to concrete, fly ash reacts with the calcium hydroxide present in the concrete to form additional cementitious materials, resulting in improved strength and durability. Fly ash increases the durability of concrete by improving its resistance to sulphate and acid attacks, reducing shrinkage and decreasing the permeability of concrete. Fly ash also enhances the workability and pumpability of concrete while reducing the heat of hydration, which reduces the risk of thermal cracking. In cold climates, fly ash helps to reduce the risk of freeze-thaw damage.
- Slag is a by-product of steel production and is used as an SCM because of its high silica and alumina content. When added to concrete, slag reacts with the calcium hydroxide present in the concrete to form additional cementitious materials, resulting in improved strength and durability. Slag increases the durability of concrete by improving its resistance to sulphate and acid attacks, reducing shrinkage and improving the strength of concrete over time. Slag also enhances the workability of concrete, reduces the heat of hydration, and improves the resistance of concrete to chloride penetration.
- Silica fume is a by-product of the production of silicon and ferrosilicon alloys and is used as an SCM because of its high silica content. When added to concrete, silica fumes react with the calcium hydroxide present in the concrete to form additional cementitious materials, resulting in improved strength and durability. Silica fume increases the durability of concrete by improving its resistance to sulphate and acid attacks, reducing permeability, and improving abrasion resistance. Silica fume also enhances the workability of concrete, reduces the heat of hydration, and improves the resistance of concrete to chloride penetration.
Overall, the use of SCMs such as fly ash, slag and silica fume can significantly improve the durability and strength of concrete in different environmental conditions. Their impact on concrete varies depending on the availability, physical and chemical properties of the specific SCM being used and proper testing and engineering analysis should be done for each mix design in order to optimise the final product.
With the global push for sustainability, how do SCMs contribute to reducing the carbon footprint of cement production?
SCMs provide an environmentally friendly alternative to traditional Portland cement by reducing the amount of clinker required to produce cement. Clinker is the main ingredient in Portland cement and is produced by heating limestone and other raw materials to high temperatures, which releases significant GHG emissions. Thus, by using SCMs, less clinker is required, thereby reducing GHG emissions, energy use and the environmental impact of cement production. Some SCMs such as fly ash and slag are by-products of other industrial processes, meaning that their use in cement production reduces waste and enhances resource efficiency. Moreover, the use of SCMs can enhance the properties of concrete, thereby increasing its durability and service life which helps to further reduce the overall embodied carbon of the structure.
In short, the use of SCMs contributes to reducing the carbon footprint of cement production by improving the efficiency of resource utilisation and reducing greenhouse gas (GHG) emissions during the production process. This has led to an increased demand for SCMs in the construction industry, as environmental concerns and sustainable development goals have become more prominent factors in the selection of building materials.
What strategies or innovations has your company adopted to ensure a consistent and reliable supply of SCMs, given their reliance on industrial by-products?
- Developing partnerships with suppliers: Many cement and concrete manufacturers establish long-term partnerships with suppliers of SCMs. These partnerships provide a reliable supply of high-quality SCMs, improve supply chain efficiency, and often provide access to new sources of SCMs.
- Advanced SCM processing techniques: Many companies are investing in advanced processing techniques to unlock new sources of high-quality SCMs. Advanced processing techniques include new separation processes, calcination techniques, and chemical activation methods.
- Alternative SCM sources: Many companies are exploring alternative SCM sources to supplement or replace traditional SCMs. Examples include agricultural by-products such as rice hull ash or sugar cane bagasse ash, which can be used in place of fly ash.
- Quality control measures: Strict quality control measures are necessary to ensure consistent quality of SCMs. Many companies use advanced testing methods, such as particle size analysis, chemical analysis, and performance testing, to validate the quality of SCM materials used in production.
- Supply chain diversification: Diversifying suppliers and SCM sources is another way to ensure a reliable supply. This reduces the risk of supply chain disruptions caused by factors such as natural disasters, market changes, or geopolitical risks.
The strategies and innovations adopted to ensure a consistent and reliable supply of SCMs include establishing long-term partnerships with suppliers, investing in advanced processing techniques, exploring alternative SCM sources, implementing strict quality control measures, and diversifying supply chains. By implementing these approaches, we ensure that use of SCMs in cement production is an effective and viable solution for reducing the environmental impact of operations
How does the use of SCMs align with your company’s broader goals around circular economy and resource efficiency?
Here are some ways in which the use of SCMs supports these goals:
- Reducing waste: The use of SCMs, such as fly ash and slag, diverts significant quantities of industrial waste from landfills, turning it into a valuable resource that can be used in construction. This helps to reduce waste and conserve natural resources.
- Reducing carbon emissions: Cement production is a significant contributor to greenhouse gas emissions, and the use of SCMs can significantly reduce the amount of cement required in concrete mixtures. This helps to reduce the carbon footprint of construction activities and move towards a low-carbon economy.
- Enhancing resource efficiency: The use of SCMs can reduce the demand for raw materials, energy, and water in the production of concrete. This not only conserves natural resources but also reduces the costs associated with the extraction, transportation and processing of these materials.
- Closing the loop: SCMs encourage closed-loop systems in the construction sector, where waste materials from one process become input materials for another. This can improve the efficiency and sustainability of the construction industry.
- Supporting sustainable design practices: The use of SCMs can support sustainable design practices by improving the durability and performance of structures while also reducing their environmental impact. This supports a circular approach to design, construction and operation of buildings and infrastructure
that improves their social, economic and environmental sustainability.
What future trends or developments do you foresee in the use of SCMs within the cement industry?
Future trends in the use of SCMs within the cement industry are likely to focus on: increased utilisation of diverse waste-derived SCMs, development of new SCM sources to address potential shortages, advanced characterisation techniques to optimise SCM blends and data-driven approaches to predict and optimise SCM usage for reduced carbon footprint and improved concrete performance; all driven by the growing need for sustainable cement production and stricter environmental regulations.
Key aspects of this trend include:
- Expanding SCM sources: Exploring a wider range of industrial byproducts and waste materials like recycled concrete aggregate, activated clays and certain types of industrial minerals as potential SCMs to reduce reliance on traditional sources like fly ash, which may become increasingly limited.
- Advanced material characterisation: Utilising sophisticated techniques to better understand the chemical and physical properties of SCMs, allowing for more precise blending and optimisation of their use in cement mixtures.
- Data-driven decision making: Implementing machine learning and big data analysis to predict the performance of different SCM combinations, allowing for real-time adjustments in cement production based on available SCM sources and desired concrete properties.
- Focus on local sourcing: Prioritising the use of locally available SCMs to reduce transportation costs and environmental impact.
- Development of new SCM processing techniques: Research into methods to enhance the reactivity and performance of less readily usable SCMs through processes like activation or modification.
- Life cycle analysis (LCA) integration: Using LCA to assess the full environmental impact of different SCMs and optimise their use to minimise carbon emissions throughout the cement production process.
- Regulatory frameworks and standards:Increased adoption of building codes and industry standards that promote the use of SCMs and set targets for reduced carbon emissions in cement production.
– Kanika Mathur

Jefferies’ Optimism Fuels Cement Stock Rally

Steel Ministry Proposes 25% Safeguard Duty on Steel Imports

India Imposes Anti-Dumping Duty on Solar Panel Aluminium Frames

Ambuja Cements, Coolbrook to Revolutionize Cement Production

UltraTech Cement Shares Surge 2.9% on Capacity Expansion

Jefferies’ Optimism Fuels Cement Stock Rally

Steel Ministry Proposes 25% Safeguard Duty on Steel Imports

India Imposes Anti-Dumping Duty on Solar Panel Aluminium Frames

Ambuja Cements, Coolbrook to Revolutionize Cement Production
